106 The Heart
Anatomy of the Heart
The heart is an organ responsible for pumping blood through the blood vessels using rhythmic contractions of cardiac muscle.
Learning Objectives
Describe the anatomy of the heart
Key Takeaways
Key Points
- The heart is a four-chambered muscular organ containing an involuntary conduction system that initiates rhythmic contractions to pump blood throughout the body.
- The heart has its own blood supply and is controlled by self-regulating nerve bundles called nodes.
- The SA and AV nodes send impulses through the Purkinje fibers that cause muscle contractions to the heart.
- The heart is composed of three layers: the epicardium (outer layer) which prevents excess expansion or movement of the heart, the myocardium (middle layer) which initiates contractions driving the cardiac cycle, and the endocardium (inner layer) that lines the cavities and valves.
- The heart contains two upper chambers called atria and two lower chambers called ventricles. The left and right sides of the heart are separated by the septum.
- Valves prevent the backflow of blood and separate the atria from the ventricle and the ventricle from the pulmonary artery or aorta.
Key Terms
- heart: A fist-sized muscular organ in the chest that pumps blood through the body using involuntary contractions of cardiac muscle.
- ventricle: One of two lower chambers of the heart that receives blood from the atria and pumps it out into pulmonary or systemic circulation, depending on side.
- myocardium: The middle of the three layers forming the wall of the heart, containing cardiac muscle tissue. Innervated by the Purkinje fibers.
The human heart is the pump for the circulatory system, and along with the circulatory system is considered to be an organ of the cardiovascular system. It consists of four chambers and pumps blood through both systemic and pulmonary circulation to enable gas exchange and tissue oxygenation. The heart is located in the thoracic cavity between the lungs and protected by the rib cage.
Structure of the Heart
The heart consists of four chambers separated into two sides. Each side contains an atria which receives blood into the heart and flows it into a ventricle, which pumps the blood out of the heart. The atria and ventricle on each side of the heart are linked together by valves that prevent backflow of blood. The wall that separates the left and right side of the heart is called the septum.
The left heart deals with systemic circulation, while the right heart deals with pulmonary circulation. The left side of the heart receives oxygenated blood from the pulmonary vein and pumps it into the aorta, while the right side of the heart receives deoxygenated blood from the vena cava and pumps it into the pulmonary vein. The pulmonary vein and aorta also have valves connecting them to their respective ventricle.
The heart has its own self-sustaining conduction system that sends nervous impulses to cardiac tissue. The sinoatrial (SA) and atrioventricular (AV) nodes are bundles of nerve fibers that form this conduction system. They are located in the left atrial wall of the heart and send nerve impulses to a large, highly specialized set of nerves called the Purkinje fibers, which in turn send those nerve impulses to the cardiac muscle tissue. These nodes can send impulses to the heart without central nervous system stimulation, but may be influenced by nervous stimulation to alter heart rate. The heart also has its own blood supply, the cardiac arteries that provide tissue oxygenation to the heart as the blood within the heart is not used for oxygenation by the heart.
Cardiac Histology
The heart is enclosed in a double-walled protective membrane called the pericardium, which is a mesothelium tissue of the thoracic cavity. The double membrane of pericardium contains pericardial fluid which nourishes the heart and prevents shock. This composite sac protects the heart, anchors it to surrounding structures, and prevents the heart from overfilling with blood.
The wall of the heart is composed of three layers of different tissues. The outer layer is called the epicardium, or visceral pericardium, since it is also the inner wall of the pericardium. The middle layer of the heart, the myocardium, and contains specialized cardiac muscle tissue responsible for contraction. Cardiac muscle tissue is distinct from skeletal or smooth muscle because it pumps involuntarily based on conduction from the AV and SA nodes. The inner layer is called the endocardium and is in contact with the blood that the heart pumps. It also merges with the inner lining of blood vessels and covers heart valves. Cardiac tissue is permanent tissue that does not heal or regenerate when damaged. As a result, is prone to scarring and enlargement due to mechanical stress and injury.
The Mammalian Heart: The position of valves ensures proper directional flow of blood through the cardiac interior. Note the difference in the thickness of the muscled walls of the atrium and the left and right ventricle.
Pericardium
The pericardium is a thick, membranous, fluid-filled sac which encloses, protects, and nourishes the heart.
Learning Objectives
Distinguish between the fibrous and serous layers of the pericardium
Key Takeaways
Key Points
- The pericardium is a mesothelium tissue of the thoracic cavity which surrounds the heart. The outer layer, the fibrous pericardium, is comprised of dense connective tissue that protects the heart, anchors it to the surrounding walls, and prevents it from overfilling.
- The inner layer of the pericardium, the serous pericardium, is further divided into two layers, an outer parietal layer and an inner visceral layer with the pericardial cavity in between the two layers.
- The serous pericardium functions to lubricate the heart with pericardial fluid, which is stored in the space between the parietal and visceral layers.
- Pericarditis is inflammation of the pericardium, often due to infection, which can constrict and put harmful pressure on the heart.
Key Terms
- fibrous pericardium: Composed of dense connective tissue which protects the heart, anchors it to the surrounding walls, and prevents the heart from overfilling with blood.
- serous pericardium: Located deeper than the fibrous pericardium, this structure contains two layers that lubricate the heart to prevent friction from occurring during heart activity.
- pericardium: A serous membrane that surrounds and protects the heart.
The pericardium is the thick, membranous, fluid-filled sac that surrounds the heart and the roots of the vessels that enter and leave this vital organ, functioning as a protective membrane. The pericardium is one of the mesothelium tissues of the thoracic cavity, along with the pleura which cover the lungs. The pericardium is composed of two layers, an outer fibrous pericardium and an inner serous pericardium.
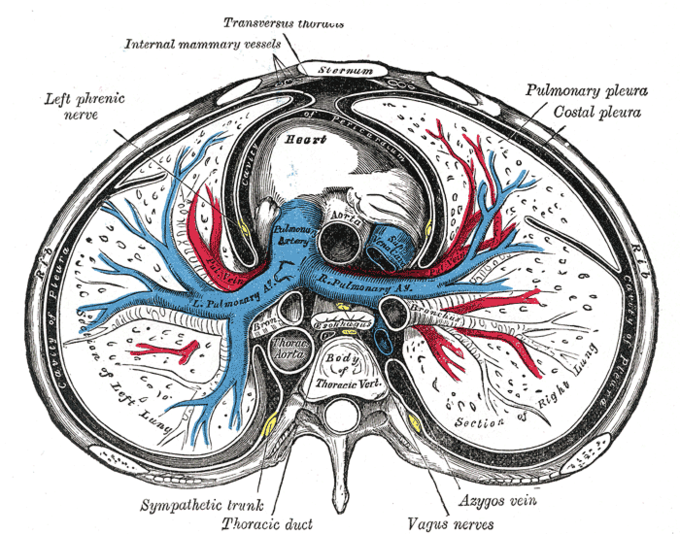
Membranes of the Thoracic Cavity: A transverse section of the thorax, showing the contents of the middle and the posterior mediastinum. The pleural and pericardial cavities are exaggerated since normally there is no space between parietal and visceral pleura and between pericardium and heart.
Fibrous Pericardium
The fibrous pericardium is the outer layer of the pericardium. It is composed of dense connective tissue which anchors the heart to the mediastinum of the chest wall. It prevents the heart from overfilling with blood and protects it from nearby infections by completely separating it from the rest of the thoracic cavity. It is continuous with the outer fibrous layer of the neighboring great blood vessels.
Serous Pericardium
The serous pericardium, the inner layer of the pericardium, is composed of two different layers. The outer layer, the parietal layer, is completely adhered to the fibrous pericardium. The inner layer is known as the visceral layer, which covers and protects the great vessels and heart. The space between the parietal and visceral layers is called the pericardial cavity.
The visceral layer is referred to as the epicardium in the areas where it is in direct contact with the heart. The space between these two serous layers, the parietal and the visceral, is the pericardial cavity, which contains pericardial fluid. The serous pericardium, with its two membranes and the fluid-filled pericardial cavity, provides protection to the heart and a lubricated sliding surface within which the heart can move in response to its own contractions and to the movement of adjacent structures such as the diaphragm and the lungs.
Function of the Pericardium
The pericardium is important because it protects the heart from trauma, shock, stress, and even infections from the nearby lungs. It supports the heart and anchors it to the medastinum so it doesn’t move within the body. The pericardium lubricates the heart and prevents it from becoming too large if blood volume is overloaded (though it will not prevent chronic heart enlargement).
Despite these functions, the pericardium is still vulnerable to problems of its own. Pericarditis is the term for inflammation in the pericardium, typically due to infection. Pericarditis is often a severe disease because it can constrict and apply pressure on the heart and work against its normal function. Pericarditis comes in many types depending on which tissue layer is infected.
Layers of the Heart Walls
The heart wall is comprised of three layers: the outer epicardium, the middle myocardium, and the inner endocardium.
Learning Objectives
Distinguish between the epicardium, myocardium, and endocardium layers of the heart wall
Key Takeaways
Key Points
- The epicardium is a thin layer of connective tissue and fat serving as an additional layer of protection for the heart. It is considered a continuation of the serous pericardium.
- The myocardium is the muscle tissue of the heart, composed of cardiac muscle cells called cardiomyocytes that receive nervous stimulation from the sinoatrial (SA) and atrioventricular (AV nodes via the Purkinje fibers.
- Cardiomyocytes are shorter than skeletal myocytes, and contain fewer nuclei. Cardiac muscle is striated.
- The endocardium is composed of endothelial cells that provide a smooth, non-adherent surface for blood collection and pumping and may help regulate contractility.
- An infection or inflammation of the endocardium is called infective endocarditis.
Key Terms
- Purkinje fibers: A bundle of nerve fibers located under the endocardium, which supply nervous impulses to the mycardium’s cardiac muscle tissues.
- endocardium: A thin serous membrane that lines the interior of the heart and valves.
- cardiomyocyte: A cardiac muscle cell (or myocyte) in the heart, which makes up the cardiac muscle tissue.
The heart wall is comprised of three layers, the epicardium (outer), myocardium (middle), and endocardium (inner). These tissue layers are highly specialized and perform different functions. During ventricular contraction, the wave of depolarization from the SA and AV nodes moves from within the endocardial wall through the myocardial layer to the epicardial surface of the heart.
Epicardium
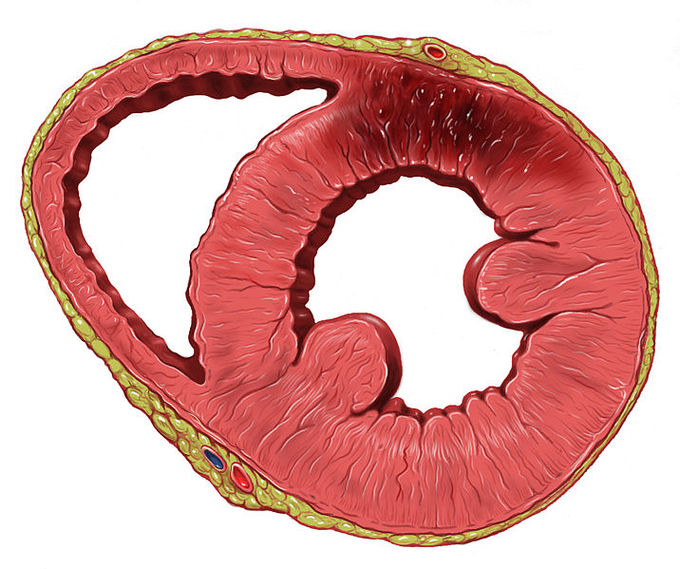
The Heart Wall: The wall of the heart is composed of three layers, the thin outer epicardium, the thick middle myocardium, and the very thin inner endocardium. The dark area on the heart wall is scarring from a previous myocardial infarction (heart attack).
The outer layer of the heart wall is the epicardium. The epicardium refers to both the outer layer of the heart and the inner layer of the serous visceral pericardium, which is attached to the outer wall of the heart. The epicardium is a thin layer of elastic connective tissue and fat that serves as an additional layer of protection from trauma or friction for the heart under the pericardium. This layer contains the coronary blood vessels, which oxygenate the tissues of the heart with a blood supply from the coronary arteries.
Myocardium
The middle layer of the heart wall is the myocardium—the muscle tissue of the heart and the thickest layer of the heart wall. It is composed of cardiac muscle cells, or cardiomyocytes. Cardiomyocytes are specialized muscle cells that contract like other muscle cells, but differ in shape. Compared to skeletal muscle cells, cardiac muscle cells are shorter and have fewer nuclei. Cardiac muscle tissue is also striated (forming protein bands) and contains tubules and gap junctions, unlike skeletal muscle tissue. Due to their continuous rhythmic contraction, cardiomyocytes require a dedicated blood supply to deliver oxygen and nutrients and remove waste products such as carbon dioxide from the cardiac muscle tissue. This blood supply is provided by the coronary arteries.
Endocardium
The inner layer of the heart wall is the endocardium, composed of endothelial cells that provide a smooth, elastic, non-adherent surface for blood collection and pumping. The endocardium may regulate metabolic waste removal from heart tissues and act as a barrier between the blood and the heart muscle, thus controlling the composition of the extracellular fluid in which the cardiomyocytes bathe. This in turn can affect the contractility of the heart.
This tissue also covers the valves of the heart and is histologically continuous with the vascular endothelium of the major blood vessels entering and leaving the heart. The Purkinje fibers are located just beneath the endocardium and send nervous impulses from the SA and AV nodes outside of the heart into the myocardial tissues.
The endocardium can become infected, a serious inflammatory condition called infective endocarditis. This and other potential problems with the endocardium may damage the valves and impair the normal flow of blood through the heart.
Chambers of the Heart
The heart has four chambers. The two atria receive blood into the heart and the two ventricles pump blood into circulation.
Learning Objectives
Describe the four chambers of the heart
Key Takeaways
Key Points
- The right and left atria are the top chambers of the heart and receive blood into the heart. The right atrium receives deoxygenated blood from systemic circulation and the left atrium receives oxygenated blood from pulmonary circulation.
- The atria do not have inlet valves, but are separated from the ventricles by valves. The atria facilitate circulation by allowing uninterrupted blood flow into the heart during ventricular systole.
- The atria have several mechanisms in place to ensure that venous blood flow isn’t impaired, such as the lack of valves and the presence of light and brief contractions during atrial systole.
- The right ventricle pumps deoxygenated blood into pulmonary circulation. The left ventricle pumps oxygenated blood into systemic circulation. The left ventricle is thicker because systemic circulation involves a much larger blood volume than pulmonary circulation.
- Systole describes contraction, when blood is pumped from the heart into circulation by the ventricles. It can also describe atrial systole, in which blood moves from the atria to the ventricles during ventricular diastole.
- Diastole describes relaxation, when blood moves into the heart while the ventricles prepare to eject that same blood during systole.
Key Terms
- diastole: The relaxation and dilation of the heart chambers between contractions, during which they fill with blood.
- systole: The rhythmic contraction of the heart by which blood is ejected from one chamber to the next, or out of the heart and into the arteries.
The heart is the complex pump of the circulatory system, pumping blood throughout the body for the purposes of tissue oxygenation and gas exchange. The heart has four chambers through which blood flows: two sets of each type of chamber (atria and ventricles), one per side, each with distinct functions. The left side of the heart deals with systemic circulation while the right side of the heart deals with pulmonary circulation.
The Atria
The atria are chambers in which blood enters the heart. They are located on the anterior end of the heart, with one atrium on each side. The right atrium receives deoxygenated blood from systemic circulation through the superior vena cava and inferior venae cavae. The left atrium receives oxygenated blood from pulmonary circulation through the left and right pulmonary veins.
Blood passively flows into the atria without passing through valves. The atria relax and dilate (expand) while they fill with blood in a process called atrial diastole. The atria and ventricles are separated by the mitral and tricuspid valves. The atria undergo atrial systole, a brief contraction of the atria that ejects blood from the atria through the valves and into the ventricles. The chordae tendinae are elastic tendons that attach to the valve from the ventricles and relax during atrial systole and ventricular diastole, but contract and close off the valve during ventricular systole.
One of the defining characteristics of the atria is that they do not impede venous flow into the heart. Atria have four essential characteristics that cause them to promote continuous venous flow:
- There are no atrial inlet valves to interrupt blood flow during atrial systole. The venous blood entering the heart has a very low pressure compared to arterial blood, and valves would require venous blood pressure to build up over a long period of time to enter the atria.
- The atrial systole contractions are incomplete and do not block flow from the veins through the atria into the ventricles. During atrial systole, blood not only empties from the atria to the ventricles, but continues to flow uninterrupted from the veins right through the atria into the ventricles.
- The atrial contractions are slight, preventing significant back pressure that would impede venous flow.
- The relaxation of the atria is coordinated to begin before the start of ventricular contraction, which also helps prevent the heart from beating too slowly.
Ventricles
The ventricles are located on the posterior end of the heart beneath their corresponding atrium. The right ventricle receives deoxygenated blood from the right atria and pumps it through the pulmonary vein and into pulmonary circulation, which goes into the lungs for gas exchange. The left ventricle receives oxygenated blood from the left atria and pumps it through the aorta into systemic circulation to supply the tissues of the body with oxygen.
The walls of the ventricles are thicker and stronger than those of the atria. The physiologic load on the ventricles, which pump blood throughout the body and lungs, is much greater than the pressure generated by the atria to fill the ventricles. Further, the left ventricle has thicker walls than the right because it pumps blood throughout the body, while the right ventricle pumps only to the lungs, which is a much smaller volume of blood.
During ventricular diastole, the ventricles relax and fill with blood. During ventricular systole, the ventricles contract, pumping blood through the semi-lunar valves into systemic circulation.
Structure of the heart: Structure diagram of a coronal section of the human heart from an anterior view. The two larger chambers are the ventricles.
Great Vessels of the Heart
Great vessels are the major vessels which directly carry blood into or out of the heart.
Learning Objectives
Describe the great vessels that carry blood to and from the heart
Key Takeaways
Key Points
- Five great vessels enter and leave the heart: the superior and inferior vena cava, the pulmonary artery, the pulmonary vein, and the aorta.
- The superior vena cava and inferior vena cava are veins that return deoxygenated blood from circulation in the body and empty it into the right atrium.
- The pulmonary artery carries deoxygenated blood from the right ventricle into the lungs for oxygenation.
- The pulmonary veins carry oxygenated blood from the lungs into the left atrium where it is returned to systemic circulation.
- The aorta is the largest artery in the body. It carries oxygenated blood from the left ventricle of the heart into systemic circulation.
- The aorta has many subdivisions that branch off into smaller arteries. These subdivisions are the ascending and descending aorta, the aortic arch, and the thoracic and abdominal aorta.
Key Terms
- pulmonary arteries: The arteries that take deoxygenated blood away from the right side of the heart and into the capillaries of the lungs for the purpose of gas exchange.
- aorta: The great artery which carries the blood from the heart into systemic circulation.
- venae cavae: The two large vessels, the superior and inferior vena cava, that bring deoxygenated blood from systemic circulation to the heart.
The human circulatory system is a double system, meaning there are two separate systems of blood flow: pulmonary circulation and systemic circulation. The adult human heart consists of two separated pumps, the right side (right atrium and ventricle,) which pumps deoxygenated blood into the pulmonary circulation, and the left side (left atrium and ventricle), which pumps oxygenated blood into the systemic circulation. Great vessels are the major vessels that carry blood into the heart and away from the heart to and from the pulmonary or systemic circuit. The great vessels collect and distribute blood across the body from numerous smaller vessels.
The Venae Cavae
The Systemic Circuit: The venae cavae and the aorta form the systemic circuit, which circulates blood to the head, extremities and abdomen.
The superior and inferior vena cava are collectively called the venae cavae. The venae cavae, along with the aorta, are the great vessels involved in systemic circulation. These veins return deoxygenated blood from the body into the heart, emptying it into the right atrium. The venae cavae are not separated from the right atrium by valves.
Superior Vena Cava
The superior vena cava is a large, short vein that carries deoxygenated blood from the upper half of the body to the right atrium. The right and left subclavian veins, jugular veins, and thyroid veins feed into the superior vena cava. The subclavian veins are significant because the thoracic lymphatic duct drains lymph fluid into the subclavian veins, making the superior vena cava a site of lymph fluid recirculation into the plasma. The superior vena cava begins above the heart.
Inferior Vena Cava
The inferior vena cava is the largest vein in the body and carries deoxygenated blood from the lower half of the body into the heart. The left and right common iliac veins converge to form the inferior vena cava at its lowest point. The inferior vena cava begins posterior to the abdominal cavity and travels to the heart next to the abdominal aorta. Along the way up the body from the iliac veins, the renal and suprarenal veins ( kidney and adrenal glands), lumbar veins (from the back), and hepatic veins (from the liver) all drain into the inferior vena cava.
The Aorta
The aorta is the largest of the arteries in systemic circulation. Blood is pumped from the left ventricle through the aortic valve into the aorta. The aorta is a highly elastic artery and is able to dilate and constrict in response to blood pressure and volume. When the left ventricle contracts to force blood through the aortic valve into the aorta, the aorta expands. This expansion provides potential energy to help maintain blood pressure during diastole, when the aorta passively contracts. Blood pressure is highest in the aorta and diminishes through circulation, reaching its lowest points at the end of venous circulation. The difference in pressure between the aorta and right atrium accounts for blood flow in the circulation, as blood flows from areas of high pressure to areas of low pressure.
Components of the Aorta
The aortic arch contains peripheral baroreceptors (pressure sensors) and chemoreceptors (chemical sensors) that relay information concerning blood pressure, blood pH, and carbon dioxide levels to the medulla oblongata of the brain. This information is processed by the brain and the autonomic nervous system mediates the homeostatic responses that involve feedback in the lungs and kidneys. The aorta extends around the heart and travels downward, diverging into the iliac arteries. The five components of the aorta are:
- The ascending aorta lies between the heart and the arch of aorta. It breaks off into the aortic sinuses, some of which form the coronary arteries.
- The arch of aorta is the peak of the aorta, which breaks off into the left carotid artery, brachiocephalic trunk, and the left subclavian artery.
- The descending aorta is the section from the arch of aorta to the point where it divides into the common iliac arteries. It is subdivided into the thoracic and abdominal aorta.
- The thoracic aorta is the part of the descending aorta above the diaphragm. It branches off into the bronchial, mediastinal, esophageal, and phrenic arteries.
- The abdominal aorta is the part of the descending aorta below the diaphragm, which divides into the iliac arteries and branches into the renal and suprarenal arteries. This part of the aorta is vulnerable to bursting and hemorrhage (aneurysm) from persistently high blood pressure.
The Pulmonary Arteries
The pulmonary arteries carry deoxygenated blood from the right ventricle into the alveolar capillaries of the lungs to unload carbon dioxide and take up oxygen. These are the only arteries that carry deoxygenated blood, and are considered arteries because they carry blood away from the heart. The short, wide vessel branches into the left and right pulmonary arteries that deliver deoxygenated blood to the respective lungs. Blood first passes through the pulmonary valve as it is ejected into the pulmonary arteries.
Pulmonary circuit: Diagram of pulmonary circulation. Oxygen-rich blood is shown in red; oxygen-depleted blood in blue.
The Pulmonary Veins
The pulmonary veins carry oxygenated blood from the lungs to the left atrium of the heart. Despite carrying oxygenated blood, this great vessel is still considered a vein because it carries blood towards the heart. Four pulmonary veins enter the left atrium. The right pulmonary veins pass behind the right atrium and superior vena cava while the left pass in front of the descending thoracic aorta. The pulmonary arteries and veins are both considered part of pulmonary circulation.
Myocardial Thickness and Function
The myocardium (cardiac muscle) is the thickest section of the heart wall and contains cardiomyocytes, the contractile cells of the heart.
Learning Objectives
Describe myocardial thickness and function in the heart
Key Takeaways
Key Points
- Coordinated contraction of cardiomyocytes causes the heart to contract and expel blood into circulation.
- The myocardium is thickest in the left ventricle, as the left ventricle must create a lot of pressure to pump blood into the aorta and throughout systemic circulation.
- Cardiac muscle has a high density of mitochondria and a large blood supply, which keep it functioning continuously.
- Sarcomeres are the basic unit of muscle tissue, composed primarily of actin and myosin myofibrils.
- The heart’s thickness may change over time and adapt to compensate for diseases, which often results in heart failure.
Key Terms
- Cardiac hypertrophy: An adaptive disease in which the walls of the heart become too thick to pump blood effectively. It is generally a complication of hypertension.
- sarcomere: The basic contractile unit of contractile muscle, which contains myofibril filaments made out of myosin and actin, the two proteins that slide past one another to cause a muscle contraction.
- cardiomyocyte: A cardiac muscle cell (myocyte) in the heart.
The myocardium, or cardiac muscle, is the thickest section of the heart wall and contains cardiomyocytes, the contractile cells of the heart. As a type of muscle tissue, the myocardium is unique among all other muscle tissues in the human body. The thickness of the myocardium determines the strength of the heart’s ability to pump blood.
Myocardial Histology
The structure of cardiac muscle shares some characteristics with skeletal muscle, but has many distinctive features of its own. Cardiomyocytes are shorter than skeletal myocytes and have fewer nuclei. Each muscle fiber connects to the plasma membrane (sarcolemma) with distinctive tubules ( T-tubule ). At these T-tubules, the sarcolemma is studded with a large number of calcium channels which allow calcium ion exchange at a rate much faster than that of the neuromuscular junction in skeletal muscle. The flux of calcium ions into the muscle cells causes stimulates an action potential, which causes the cells to contract.
Cardiac muscle, like skeletal muscle, is comprised of sarcomeres, the basic, contractile units of muscle. Sarcomeres are composed of long, fibrous proteins that slide past each other when the muscles contract and relax. Two of the important proteins found in sarcomeres are myosin, which forms the thick filament, and actin, which forms the thin filament. Myosin has a long, fibrous tail and a globular head that binds to actin. The myosin head also binds to ATP, the source of energy for cellular metabolism, and is required for the cardiomyocytes to sustain themselves and function normally. Together, myosin and actin form myofibril filaments, the elongated, contractile threads found in muscle tissue. Cardiac muscle and skeletal muscle both contain the protein myoglobin, which stores oxygen.
Cardiac muscle is adapted to be highly resistant to fatigue. Cardiomyocytes have a large number of mitochondria, enabling continuous aerobic respiration. Cardiac muscle also has a large blood supply relative to its size, which provides a continuous stream of nutrients and oxygen while providing ample removal of metabolic waste.
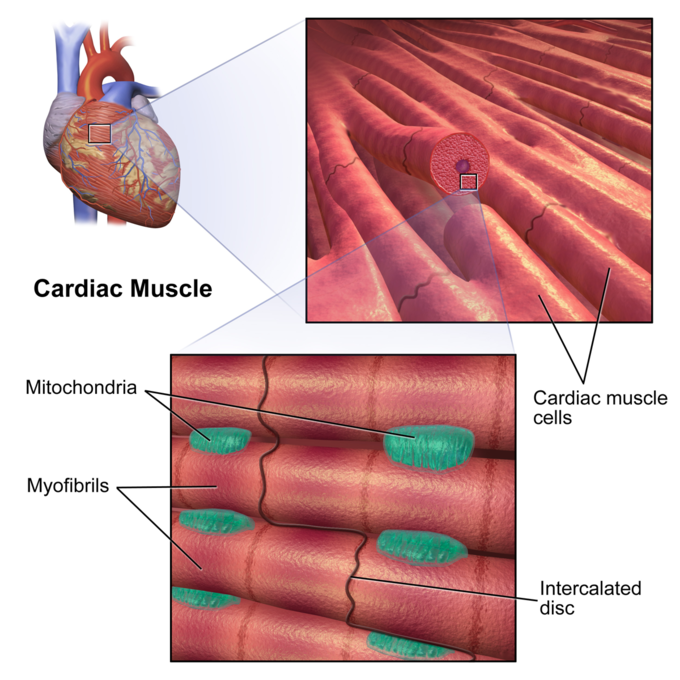
Cardiac Muscle: The tissue structure of cardiac muscle contains sarcomeres that are made of myofibrils with intercalated disks, that contain cardiomyocytes and have many mitocondria.
Myocardial Thickness
The myocardium has variable levels of thickness within the heart. Chambers of the heart with a thicker myocardium are able to pump blood with more pressure and force compared to chambers of the heart with a thinner myocardium. The myocardium is thinnest within the atria, as these chambers primarily fill through passive blood flow. The right ventricle myocardium is thicker than the atrial myocardium, as this muscle must pump all blood returning to the heart into the lungs for oxygenation. The myocardium is thickest in the left ventricle, as this chamber must create substantial pressure to pump blood into the aorta and throughout systemic circulation.
The thickness of the myocardium may change in some individuals as a compensatory adaptation to disease, either thickening and becoming stiff or becoming thinner and flabby. Cardiac hypertrophy is a common result of hypertension (high blood pressure) in which the cells of the myocardium enlarge as an adaptive response to pumping against the higher pressure. Eventually, hypertrophy may become so severe that heart failure occurs when the heart becomes so stiff that it can no longer pump blood. A flabby heart is typically the result of myocardial infections, in which the heart muscle becomes so weak that it cannot efficiently pump blood, which also leads to heart failure.
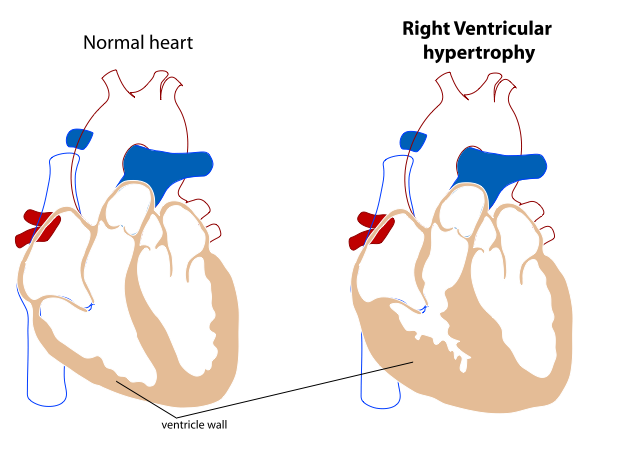
Right Ventricular Hypertrophy: If the heart adapts to become too thick, it will not be able to pump blood as efficiently, and heart failure may occur.
Fibrous Skeleton of the Heart
The cardiac skeleton, also known the heart’s fibrous skeleton, consists of dense connective tissue in the heart that separates the atria from the ventricles.
Learning Objectives
Describe composition and function of the heart’s fibrous skeleton
Key Takeaways
Key Points
- The cardiac skeleton consists of four bands of dense connective tissue, called fibrous rings, that surround the base of the pulmonary trunk, aorta, and mitral and tricuspid valves.
- The heart ‘s fibrous skeleton stops the flow of electrical currents between the chambers of the heart so that it only flows through the atrioventricular (AV) bundle. This causes a delay in depolarization so that the ventricles contract after they fill with blood.
- The AV bundle is a bundle of electrically-connected cardiomyocytes that transmit impulses from the AV node to the apex of the heart. It is located in the center of the cardiac skeleton.
- The cardiac skeleton consists mainly of the protein collagen, a glycoprotein found in all connective tissues.
Key Terms
- collagen: A glycoprotein that forms elongated fibers, usually found in the extracellular matrix of connective tissue.
- fibrous rings: Four dense bands of tough elastic tissue that encircle the bases of the valves of the heart.
The cardiac skeleton, or fibrous skeleton of the heart, is the structure of dense connective tissue that separates the atria from the ventricles. The fibrous skeleton provides critical support for the heart and separates the flow of electrical impulses through the heart.
Fibrous Ring Structure
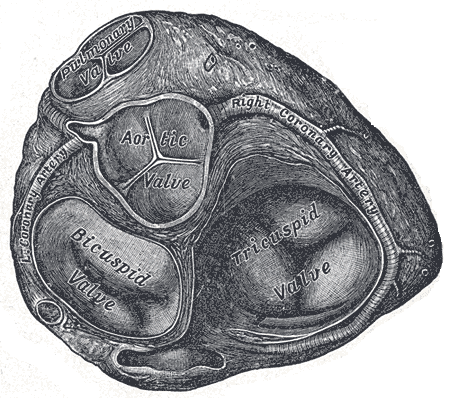
Fibrous Rings of the Heart: Transverse section of the heart showing the fibrous rings surrounding the valves.
The primary structure of cardiac skeleton consists of four dense bands of tough elastic tissue called fibrous rings that encircle the bases of the heart valves. The fibrous skeleton is composed primarily of collagen, a protein found in every type of connective tissue in the human body. There are four fibrous rings:
- The aortic ring encircles the aortic valve. It provides support for the aortic valve so that it is open, yet does not have backflow.
- The pulmonary ring encircles the pulmonary valve. Similar to the aortic ring, it provides structural support for the pulmonary valve.
- The left fibrous ring encircles the bicuspid valve. This ring is the thickest and strongest of all the fibrous rings due to the thickness of the left ventricle, which requires more structural support than the other chambers of the heart. It also surrounds the coronary arteries and AV node.
- The right fibrous ring encircles the tricuspid valve. It also surrounds the coronary arteries and AV node.
The fibrous skeleton provides a great amount of structural and functional support for the valves of the heart by enabling them to stay open and provides a point of attachment for the valves to the wall of the heart.
Electrical Functions
The fibrous skeleton of the heart acts as an insulator for the flow of electrical current across the heart. It stops the flow of electricity between the different chambers of the heart so that electrical impulses do not flow directly between the atria and ventricles. The sinoatrial (SA) node lies on the top of the heart, while the AV node is located at the center of the fibrous skeleton, the only path by which electricity can flow through the heart.
This electrical separation is essential for cardiac function, because electrical impulses flow from the top of the heart to the bottom of the heart. The separation allows the AV node and AV bundle to delay the wave of depolarization so that the atria can contract and assist in ventricular filling before the ventricles themselves depolarize and contract. Without the fibrous skeleton of the heart, the heart’s ability to pump blood would be considerably less efficient since the ventricles would contract before filled to capacity. The fibrous skeleton of the heart also protects against cardiac arrhythmias.