89 Mechanisms of Hormone Action
Direct Gene Activation and the Second-Messenger System
Nuclear receptors function as transcription factors because they can bind to DNA and regulate gene expression.
Learning Objectives
Distinguish between the hormone mechanisms of direct gene activation and the second-messenger system
Key Takeaways
Key Points
- Receptors that can directly influence gene expression are termed nuclear receptors.
Type I nuclear receptors (found in cytosol) are modified to translocate to the nucleus upon hormone binding. - Type II nuclear receptors remain in the nucleus where they often create a complex with co-repressor proteins, which are released upon hormone binding.
- Secondary messengers relay signals from receptors on the cell surface to the target molecules.
- The secondary messenger systems bind hormones to a receptor that causes a cascade of changes that leads to actions.
Key Terms
- nuclear receptor: A class of proteins found within cells that are responsible for sensing steroid and thyroid hormones and certain other molecules, as well as to influence gene expression upon activation.
- secondary messenger: Molecules that relay signals from receptors on the cell surface to target molecules inside the cell, in the cytoplasm or nucleus.
- hormone response element: A short sequence of DNA within the promoter of a gene that is able to bind a specific hormone receptor complex and therefore regulate gene expression.
Hormones can alter cell activity by binding with a receptor. Receptors can either directly influence gene expression and thus cell activity, or induce a secondary signaling cascade that will in turn influence cell activity.
Direct Gene Activation
Receptors that can directly influence gene expression are termed nuclear receptors. Located within the cytosol or nucleus, nuclear receptors are the target of steroid and thyroid hormones that are able to pass through the cell membrane. Nuclear receptors can bind directly to DNA to regulate specific gene expressions and are, therefore, classified as transcription factors.
Nuclear receptors can be classified into two broad classes according to their mechanism of action and their sub-cellular distribution in the absence of ligand. Type I nuclear receptors are located in the cytosol. Upon binding to a hormone the receptor and hormone translocate into the nucleus, and bind to specific sequences of DNA known as hormone response elements (HREs).
Type II receptors are retained in the nucleus. In the absence of ligand, type II nuclear receptors often form a complex with co-repressor proteins. Hormone binding to the nuclear receptor results in dissociation of the co-repressor and the recruitment of co-activator proteins.
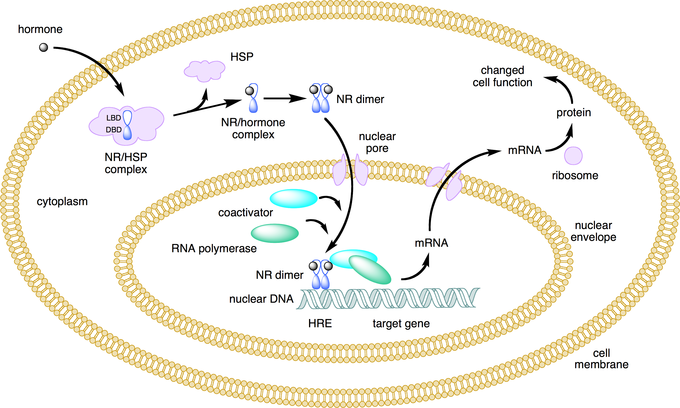
Lipid soluble hormones directly regulate gene expression: This figure depicts the mechanism of a class I nuclear receptor (NR) that, in the absence of ligand, is located in the cytosol. Hormone binding to the NR triggers translocation to the nucleus, where the NR binds to a specific sequence of DNA known as a hormone response element (HRE).
Secondary Messengers
For lipophobic hormones that cannot pass the cellular membrane, activity is mediated and amplified within a cell by the action of second messenger mechanisms (molecules that relay signals from receptors on the cell surface to target molecules inside the cell in the cytoplasm or nucleus).
Most hormone receptors are G protein-coupled receptors. Upon hormone binding, the receptor undergoes a conformational change and exposes a binding site for a G-protein. The G-protein is bound to the inner membrane of the cell and consists of three sub-units: alpha, beta, and gamma.
Upon binding to the receptor, it releases a GTP molecule, at which point the alpha sub-unit of the G-protein breaks free from the beta and gamma sub-units and is able to move along the inner membrane until it contacts another membrane-bound protein: the primary effector.
The primary effector then has an action, which creates a signal that can diffuse within the cell. This signal is called the secondary messenger. The secondary messenger may then activate a secondary effector, whose effects depend on the particular secondary messenger system.
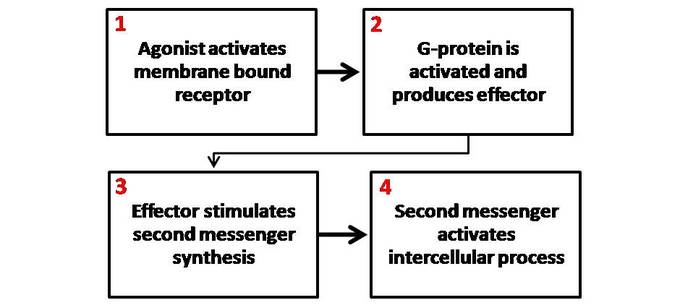
Second messenger mechanisms: General schematic of second messenger generation following activation of membrane bound receptors. 1. The agonist activates the membrane-bound receptor. 2. G-protein is activated and produces an effector. 3. The effector stimulates a second messenger synthesis. 4. The second messenger activates an intercellular process.
Target Cell Specificity
Hormones target a limited number of cells (based on the presence of a specific receptor) as they circulate in the bloodstream.
Learning Objectives
Describe target cell specificity in the endocrine system
Key Takeaways
Key Points
- Target cells are cells that are receptive to a secreted hormone.
- Target cell activation is
dependent on three factors; the hormone levels in the blood, the receptor levels on the target cell, and hormone–receptor affinity.
Key Terms
- target cell: A cell that is receptive to a secreted hormone.
Examples
An XY fetus will develop along a female pathway if the target cells fail to respond to androgen. This androgen insensitivity occurs when the receptors on the target cells are unable to accept the hormone due to an impairment in receptor shape.
In endocrinology, target cells can refer to the cells where hormones have an effect. Target cells are capable of responding to hormones because they display receptors to which the circulating hormone can bind. In this way, hormones only affect a limited number of cells even though they are transported in the bloodstream throughout the body.
Target cell activation is dependent on three factors:
- The levels of hormone in the blood.
- The relative number of hormone receptors on the target cell.
- The hormone–receptor affinity.
Modulation of these factors can control target cell response. For example, after receptor stimulation the signaling target cell often sends feedback to the hormone-secreting tissue to down-regulate hormone expression.
Additionally, the target cell can up or down-regulate receptor expression to make it more or less sensitive to the same hormone. Finally, hormone–receptor affinity can be altered by the expression of associated inhibitory or co-activating factors.
In some instances, alterations of receptor structure due to a genetic mutation can lead to a reduction in hormone–receptor affinity, as in the case of androgen insensitivity.
Onset, Duration, and Half-Life of Hormone Activity
A hormone’s half-life and duration of activity are limited and vary from hormone to hormone.
Learning Objectives
Distinguish between a hormone’s half-life and its duration of activity
Key Takeaways
Key Points
- The hormone receptors are dynamic structures that vary in number and sensitivity, that depend on the levels of the stimulating hormone.
- The blood levels of hormones reflect a balance between secretion and degradation/excretion by the liver and kidneys.
- The biological half- life of a hormone is the time it takes for the hormone to lose half of its physiological activity.
- The duration of hormone activity refers to the duration of altered cellular behavior triggered by hormone binding.
Key Terms
- hormone receptor: A molecule that binds to a specific hormone that triggers alterations in cell activity.
- half-life: The time it takes for a substance (drug, radioactive nuclide, or other) to lose half of its pharmacological, physiological, or radiological activity.
Examples
Vitamin D is a hormone that has a half-life of one to two months. If one obtains vitamin D solely through sun (UVB) exposure during the summer months, serum vitamin D levels will be critically low by late winter. This is one reason why current recommendations are to take vitamin D supplements in order to maintain serum vitamin D levels throughout the year.
The number of hormone molecules available for complex formation is usually the key factor that determines the level at which signal transduction pathways are activated. The number of hormone molecules that are available is determined by the concentration of circulating hormones.
Half-Life
The blood levels of hormones reflect a balance between synthesis/secretion and degradation/excretion. The liver and kidneys are the major organs that degrade hormones with breakdown products excreted in urine and feces.
A hormone’s half-life and duration of activity are limited and vary from hormone to hormone. For instance, the biological half-life of luteinizing hormone is 20 minutes, which is shorter than that of a follicle-stimulating hormone (three to four hours), and of human chorionic gonadotropin (24 hours).
A biological half-life or elimination half-life is the time it takes for a substance such as a hormone or drug to lose half of its pharmacologic or physiologic activity. In a medical context, half-life may also describe the time it takes for the blood plasma concentration of a substance to halve (plasma half-life) its steady-state.
The relationship between the biological and plasma half-lives of a substance can be complex, due to factors including their accumulation in tissues, active metabolites, and receptor interactions.
Duration
The duration of hormone activity refers to the duration of events that were stimulated by hormone-receptor binding. While typically relatively short and measured in minutes or hours, certain events, such as the onset of puberty, are much longer lasting.
Hormone levels during menstrual cycle: This image depicts the levels of certain hormones during the menstrual cycle (B), as they correspond to follicular growth and ovulation (A). 1. Follicle-stimulating hormone 2. Estrogen 3. Luteinizing hormone 4. Progesterone.