136 Mechanics of Breathing
Pressure Changes During Pulmonary Ventilation
Ventilation is the rate at which gas enters or leaves the lung.
Learning Objective
Differentiate among the types of pulmonary ventilation: minute, alveolar, dead space
Key Takeaways
Key Points
- Ventilation is the rate at which gas enters or leaves the lung.
- The three types of ventilation are minute ventilation, alveolar ventilation, and dead space ventilation.
- The alveolar ventilation rate changes according to the frequency of breath, tidal volume, and amount of dead space.
- PA refers to alveolar partial pressure of a gas, while Pa refers to the partial pressure of that gas in arterial blood.
- Gas exchange occurs from passive diffusion because PAO2 is greater than PaO2 in deoxygenated blood.
Key Terms
- ventilation: The bodily process of breathing, the inhalation of air to provide oxygen, and the exhalation of spent air to remove carbon dioxide.
- partial pressure: The pressure exerted by a gas, either in air or dissolved, that indicates the concentration of that gas.
The Types of Ventilation Rates
In respiratory physiology, the ventilation rate is the rate at which gas enters or leaves the lung. Ventilation is generally expressed as volume of air times a respiratory rate.
The volume of air can refer to tidal volume (the amount inhaled in an average breath) or something more specific, such as the volume of dead space in the airways. The three main types of ventilation rates used in respiratory physiology are:
- Minute ventilation (VE): The amount of air entering the lungs per minute. It can be defined as [latex]\text{VE}=\text{Tidal Volume}\times\text{Breaths Per Minute}[/latex]
- Alveolar ventilation (VA): The amount of gas per unit of time that reaches the alveoli and becomes involved in gas exchange. It is defined as [latex]\text{VA}=\left(\text{Tidal Volume}-\text{Dead Space Volume}\right)\times\text{Respiratory Rate}[/latex]
- Dead space ventilation (VD): The amount of air per unit of time that is not involved in gas exchange, such as the air that remains in the conducting zones. It is defined as [latex]\text{VD}=\text{Dead Space Volume}\times\text{Respiratory Rate}[/latex].
Additionally, minute ventilation can be described as the sum of alveolar and dead space ventilation, provided that the respiratory rate used to derive them is in terms of breaths per minute.
The three types of ventilation are mathematically linked to one another, so changes in one ventilation rate can cause the change of the other. This is most apparent in changes of the dead space volume. Breathing through a snorkeling tube and having a pulmonary embolism both increase the amount of dead space volume (through anatomical versus alveolar dead space respectively), which will reduce alveolar ventilation.
Alveolar ventilation is the most important type of ventilation for measuring how much oxygen actually gets into the body, which can initiate negative feedback mechanisms to try and increase alveolar ventilation despite the increase in dead space. In particular, the body will generally attempt to combat increased dead space by raising the frequency of breaths to try and maintain sufficient levels of alveolar ventilation.
Partial Pressure of Gasses
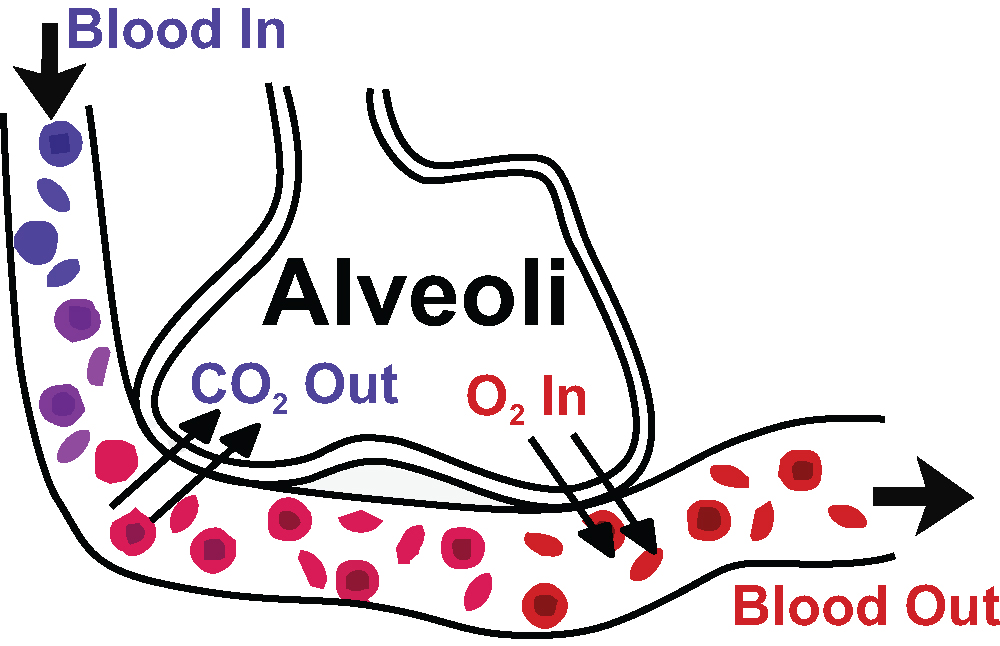
When gasses dissolve in the bloodstream during ventilation, they are generally described by the partial pressure of the gasses. Partial pressure more specifically refers to the relative concentration of those gasses by the pressure they exert in a dissolved state.
In respiratory physiology, PAO2 and PACO2,refer to the partial pressures of oxygen and carbon dioxide in the alveoli.
PaO2 and PaCO2 refer to the partial pressures of oxygen and carbon dioxide within arterial blood. Differences in partial pressures of gasses between the alveolar air and the blood stream are the reason that gas exchange occurs by passive diffusion.
Under normal conditions, PAO2 is about 100 mmHg, while PaO2 is 80–100 mmHg in systemic arteries, but 40–50 mmHg in the deoxygenated blood of the pulmonary artery going to the lungs.
Recall that gasses travel from areas of high pressure to areas of low pressure, so the greater pressure of oxygen in the alveoli compared to that of the deoxygenated blood explains why oxygen can passively diffuse into the bloodstream during gas exchange.
Conversely, PACO2 is 35 mmHg, while PaCO2 is about 40–45 mmHG in systemic arteries and 50 mmHg in the pulmonary artery. The partial pressure, and thus concentration of carbon dioxide, is greater in the in the capillaries of the alveoli compared to the alveolar air, so carbon dioxide will passively diffuse from the bloodstream into the alveoli during gas exchange.
Additionally, because PaCO2 is an indicator of the concentration of carbon dioxide in arterial blood, it can be used to measure blood pH and identify cases of respiratory acidosis and alkalkosis.
Inspiration
Inhalation is the flow of air into an organism that is due to a pressure difference between the atmosphere and alveolus.
Learning Objective
Outline the mechanics of inspiration
Key Takeaways
Key Points
- In humans, inspiration is the flow of air into an organism from the external environment, through the airways, and into the alveoli.
- Inhalation begins with the onset of a contraction of the diaphragm, which results in expansion of the thoracic and pleural cavities and a decrease in pressure (also called an increase in negative pressure).
- There are many accessory muscles involved in inhalation—such as external intercostal muscles, scalene muscles, the sternocleidomastoid muscle, and the trapezius muscle.
- Breathing only with the accessory muscles instead of the diaphragm is considered inefficient, and provides much less air during inhalation.
- The negative pressure in the pleural cavity is enough to hold the lungs open in spite of the inherent elasticity of the tissue. The thoracic cavity increases in volume causing a drop in the pressure (a partial vacuum) within the lung itself.
- As long as pressure within the alveoli is lower than atmospheric pressure, air will continue to move inwardly, but as soon as the pressure is stabilized air movement stops.
Key Terms
- inspiration: The drawing of air into the lungs, accomplished in mammals by elevation of the chest walls and flattening of the diaphragm.
- accessory muscles: Muscles that help expand small parts of the thoracic cavity, either working in addition to the diaphragm or substituting for it if the diaphragm becomes injured.
- intrapleural pressure: The pressure inside the pleural cavity, which is negative compared to outside air and becomes even more negative during inspiration.
Inspiration refers to inhalation—it is the flow of the respiratory current into an organism. In humans it is the movement of ambient air through the airways and into the alveoli of the lungs.
The Process of Inspiration
Inspiration begins with the contraction of the diaphragm, which results in expansion of the thoracic cavity and the pleural cavity. The pleural cavity normally has a lower pressure compared to ambient air (–3 mmHg normally and typically –6 mmHg during inspiration), so when it expands, the pressure inside the lungs drops.
Pressure and volume are inversely related to each other, so the drop in pressure inside the lung increases the volume of air inside the lung by drawing outside air into the lung. As the volume of air inside the lung increases, the lung pushes back against the expanded pleural cavity as a result of the drop in intrapleural pressure (pressure inside the pleural cavity).
The force of the intrapleural pressure is even enough to hold the lungs open during inpiration despite the natural elastic recoil of the lung. The alveolar sacs also expand as a result of being filled with air during inspiration, which contributes to the expansion inside the lung.
Eventually, the pressure inside the lung becomes less negative as the volume inside the lung increases and, when pressure and volume stabilize, air movement stops, inspiration ends, and expiration (exhalation) will begin. Deeper breaths have higher tidal volumes and require a greater drop in intrapleural pressure compared to shallower breaths.
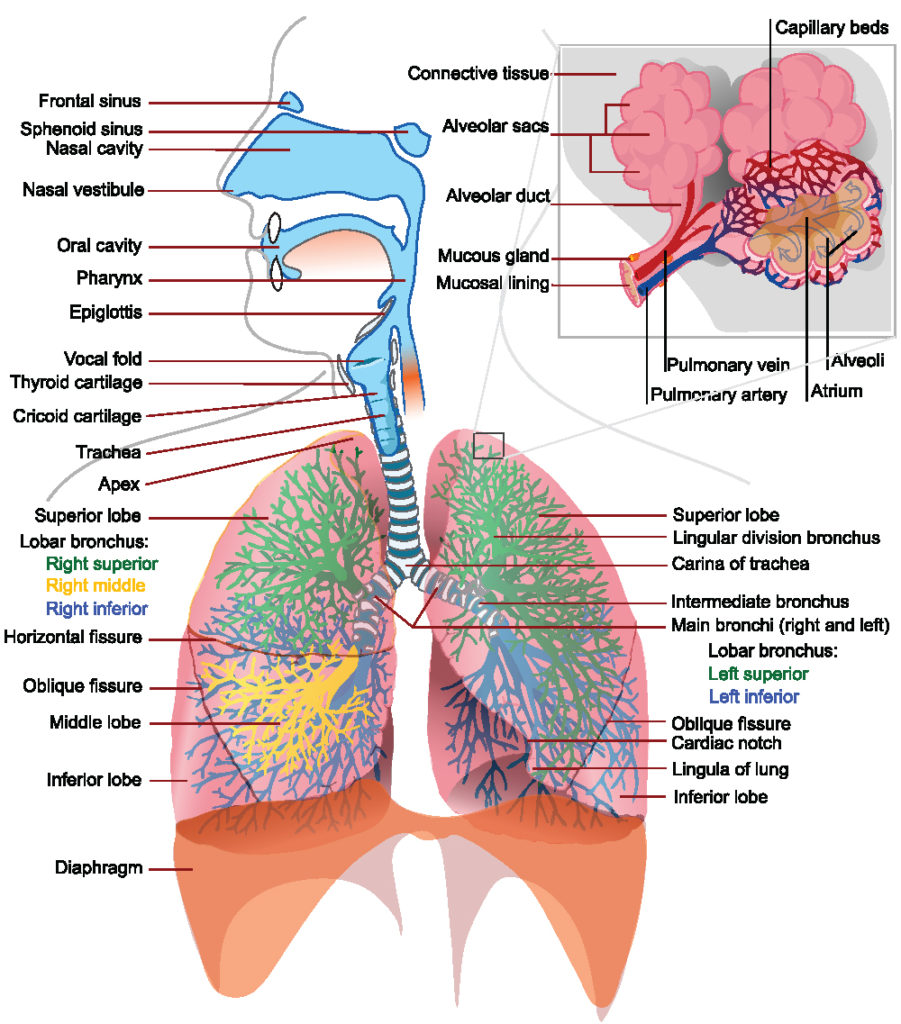
Accessory Muscles of Inspiration
The diaphragm is the primary muscle involved in breathing, however several other muscles play a role in certain circumstances. These muscles are referred to as accessory muscles of inhalation.
- External intercostal muscles: Muscles located between the ribs that help the thoracic cavity and pleural cavity expand during quiet and forced inspiration.
- Scalene muscles: Muscles in the neck that lift the upper ribs (and thoracic cavity around the upper ribs) to help with breathing. They provide a mechanism for inspiration when the diaphragm is injured and can’t contract normally.
- Sternocleidomastoid muscle: Muscles that connect the sternum to the neck and allow for rotation and turning of the head. They can lift the upper ribs like the scalene muscles can.
- Trapezius muscle: Muscles in the shoulders that retract the scapula and expand the upper part of the thoracic cavity.
The accessory muscles assist breathing by expanding the thoracic cavity in a similar way to the diaphragm. However, they expand a much smaller part of the thoracic cavity compared to diaphragm. Therefore they should not be used as the primary mechanism of inhalation, because they take in much less air compared to the diaphragm resulting in a much lower tidal volume.
For example, singers need a lot of air to support the powerful voice production needed for singing. A common problem in novice singers is breathing with the accessory muscles of the neck, shoulder, and ribs instead of the diaphragm, which gives them a much smaller air supply than what is needed to sing properly.
Expiration
Exhalation (or expiration) is the flow of the respiratory current out of the organism.
Learning Objective
Outline the mechanics of expiration
Key Takeaways
Key Points
- In humans, exhalation is the movement of air out of the bronchial tubes, through the airways, to the external environment during breathing.
- Exhalation is a passive process because of the elastic properties of the lungs.
- During forced exhalation, internal intercostal muscles which lower the rib cage and decrease thoracic volume while the abdominal muscles push up on the diaphragm which causes the thoracic cavity to contract.
- Relaxation of the thoracic diaphragm causes contraction of the pleural cavity which puts pressure on the lungs to expel the air.
- Brain control of exhalation can be broken down into voluntary control and involuntary control.
Key Terms
- Intercostal muscles: Intercostal muscles are several groups of muscles that run between the ribs, and help form and move the chest wall.
- exhalation: The act or process of exhaling, or sending forth in the form of steam or vapor; evaporation.
Expiration, also called exhalation, is the flow of the respiratory current out of the organism. The purpose of exhalation is to remove metabolic waste, primarily carbon dioxide from the body from gas exchange. The pathway for exhalation is the movement of air out of the conducting zone, to the external environment during breathing.
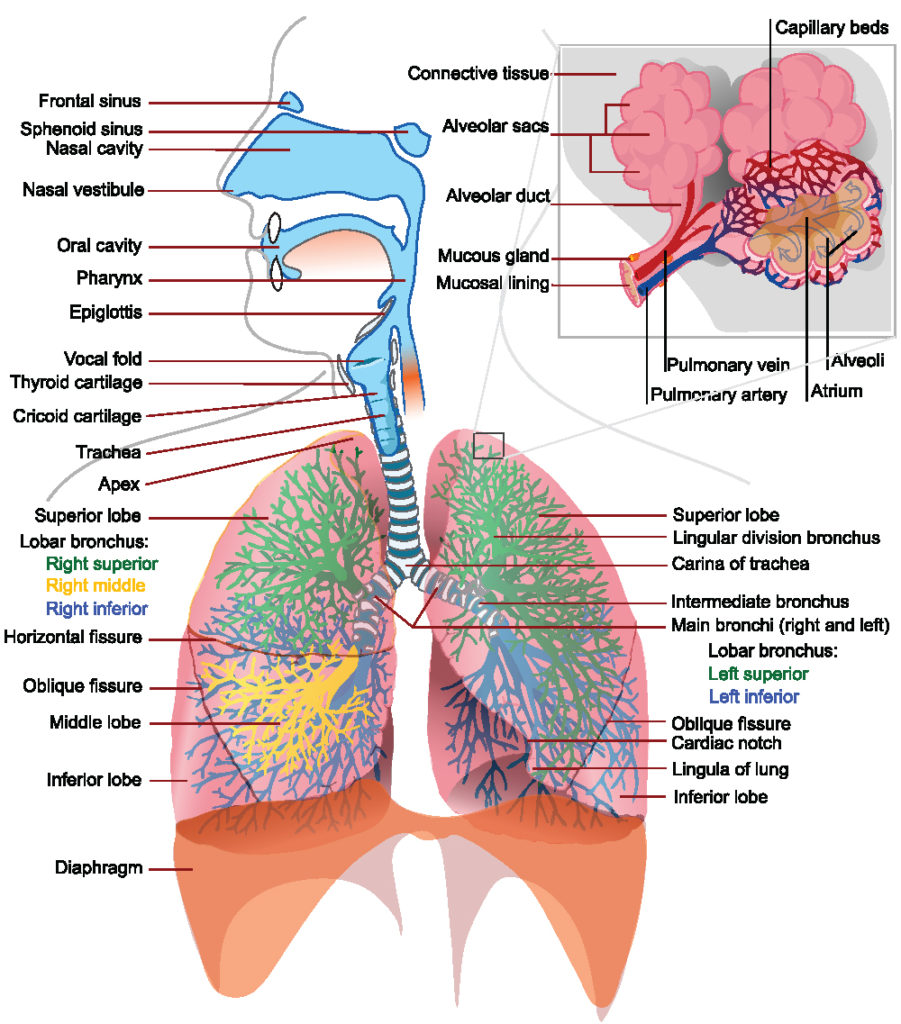
The Process of Expiration
Expiration is typically a passive process that happens from the relaxation of the diaphragm muscle (that contracted during inspiration). The primary reason that expiration is passive is due to the elastic recoil of the lungs. The elasticity of the lungs is due to molecules called elastins in the extracellular matrix of lung tissues and is maintained by surfactant, a chemical that prevents the elasticity of the lungs from becoming too great by reducing surface tension from water. Without surfactant the lungs would collapse at the end of expiration, making it much more difficult to inhale again. Because the lung is elastic, it will automatically return to its smaller size as air leaves the lung.
Exhalation begins when inhalation ends. Just as the plueral cavity’s increased negative pressure leads to air uptake during inhalation, the pleural cavity will contract during the exhalation (due to relaxation of the diaphragm),which exerts pressure on the lungs and causes the pressure inside the cavity to be less negative. An increase in pressure leads to a decrease in volume inside the lung, and air is pushed out into the airways as the lung returns to its smaller size. The pleural cavity is so important to breathing because it’s pressure changes the volume of the lungs, and it provides a friction-less space for the lung to expand and contract against during breathing.
While expiration is generally a passive process, it can also be an active and forced process. There are two groups of muscles that are involved in forced exhalation.
- Internal Intercostal Muscles: Muscles of the ribcage that help lower the ribcage, which pushes down on the thoracic cavity, causing forced exhalation. Note that these are not the same as the external intercostal muscles involved in inspiration.
- Abdominal Muscles: Any number of muscles in the abdomen that exert pressure on the diaphragm from below to expand it, which in turn contracts the thoracic cavity, causing forced exhalation.
This happens due to elastic properties of the lungs, as well as the internal intercostal muscles that lower the rib cage and decrease thoracic volume. As the thoracic diaphragm relaxes during exhalation it causes the tissue it has depressed to rise superiorly and put pressure on the lungs to expel the air.
Control of Expiration
Expiration can be either voluntary or involuntary in order to serve different purposes for the body. These two types of expiration are controlled by different centers within the body.
Voluntary expiration is actively controlled. It is generally defined by holding air in the lungs and releasing it at a fixed rate, which enables control over when and how much air to exhale. It is required for voice production during speech or singing, which requires very specific control over air, or even simpler activities, like blowing out a candle on one’s birthday. The nervous system component that controls voluntary expiration is the motor cortex (the ascending respiratory pathway), because it controls muscle movements, but this pathway isn’t fully understood, and there are many other possible sites in the brain that may also be involved.
Involuntary expiration is not under conscious control, and is an important component for metabolic function. Examples include breathing during sleep or meditation. Changes in breathing patterns may also occur for metabolic reasons, such as through increased breathing rate in people with acidosis from negative feedback. The principle neural control center for involuntary expiration consists of the medulla oblongata and the pons, which are located in the brainstem directly beneath the brain. While these two structures are involved in neural respiratory control, they also have other metabolic regulatory functions for other body systems, such as the cardiovascular system.
Breathing Patterns
Breathing is an autonomic process that moves air in and out of the lungs.
Learning Objective
Describe the process of breathing in humans
Key Takeaways
Key Points
- Breathing patterns consist of tidal volume and respiratory rate in an individual.
- An average breathing pattern is 12 breaths per minute and 500 mL per breath.
- Eupnea is normal breathing at rest.
- There are types of altered breathing patterns that are symptoms of many diseases.
- Altered breathing patterns refer to changes in respiratory rate or amount of air exchanged during breathing, and do not always indicate changes in alveolar ventilation.
- The mechanism of generation of the ventilatory pattern involves the integration of neural signals by respiratory control centers in the medulla and pons.
Key Terms
- altered breathing patterns: Abnormal breathing patterns that indicate typically indicate either too fast or too slow respiratory rate or too much or too little tidal volume.
- tidal volume: The amount of air displaced or exchanged in a single breath.
Breathing patterns refer to the respiratory rate, which is defined as the frequency of breaths over a period of time, as well as the amount of air cycled during breathing (tidal volume). Breathing patterns are an important diagnostic criteria for many diseases, including some which involve more than the respiratory system itself.
Characteristics of the Breathing Patterns
The respiratory rate is frequency of breaths over time. The time period is variable, but usually expressed in breaths per minute because it that time period allows for estimation of minute ventilation. During normal breathing, the volume of air cycled through inhalation and exhalation is called tidal volume (VT), and is the amount of air exchanged in a single breath. Tidal volume multiplied by the respiratory rate is minute ventilation, which is one of the most important indicators of lung function. In an average human adult, the average respiratory rate is 12 breaths per minute, with a tidal volume of .5 liters and a minute ventilation of 6 liters per minute, though these numbers vary from person to person. Infants and children have considerably higher respiratory rates than adults.
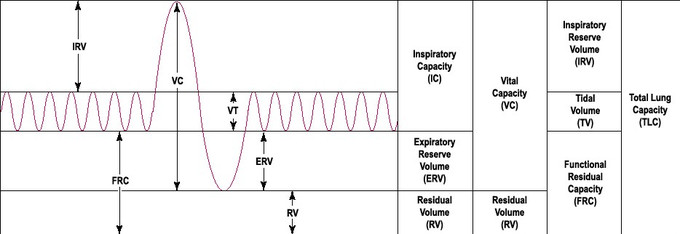
The respiratory rate is controlled by involuntary processes of the autonomic nervous system. In particular, the respiratory centers of the medulla and the pons control the overall respiratory rate based on a variety of chemical stimuli from within the body. The hypothalamus can also influence the respiratory rate during emotional and stress responses.
Normal and Altered Breathing Patterns
Eupnea is the term for the normal respiratory rate for an individual at rest. Several other terms describe abnormal breathing patterns that are indicative of symptoms of many diseases, many of which aren’t mainly respiratory diseases. Some of the more common terms for altered breathing patterns include:
- Dyspnea: commonly called shortness of breath. It describes dramatically decreased tidal volume and sometimes increased respiratory rate, leading to a sensation of breathlessness. It is a common symptom of anxiety attacks, pulmonary embolisms, heart attacks, and emphysema, among other things.
- Hypernea: refers to increased volume of air cycled to meet the body’s metabolic needs, which may or may not involve a change in frequency of breathing. It is a symptom of exercise and adjustment to high altitude, which are generally not problematic, but can also be seen in those with anemia or septic shock, which is problematic.
- Tachypnea: describes increased respiratory rate. Often a symptom of carbon monoxide poisoning or pnuemonia.
- Bradypnea: describes decreased respiratory rate. Often a symptom of hypertension, heart arryhmias, or slow metabolic rate from hypothyroidism.
- Apnea: Transient stopped breathing that begins again soon afterwards. It is the main symptom of sleep apnea, in which breathing temporarily stops during sleep.
These terms all describe an altered breathing pattern through increased or decreased (or stopped) tidal volume or respiratory rate. It is important to distinguish these terms from hyperventilation and hypoventilation, which refer to abnormalities in alveolar gas exchange (and thus blood pH) instead of an altered breathing pattern, but they may be associated with an altered breathing pattern. For example dyspnea or tachypnea often occur together with hyperventilation during anxiety attacks, though not always.