107 Circulation and Heart Valves
Heart Circulation
Coronary circulation is the circulation of blood in the blood vessels of the heart.
Learning Objectives
Describe circulation of blood in the heart
Key Takeaways
Key Points
- The vessels that supply blood to the myocardium are called coronary arteries and coronary veins.
- The left and right coronary arteries branch off into smaller arteries, such as the important left anterior descending (LAD) coronary artery.
- The vessels that deliver oxygen-rich blood to the myocardium are known as coronary arteries. The vessels that remove the deoxygenated blood from the heart muscle are known as cardiac veins.
- Most tissue perfusion in the heart occurs when the coronary arteries open during diastole.
- Failure of the coronary arteries to provide sufficient blood supply to the heart can lead to ischemia, angina, and myocardial infarction.
- Norepinephrine will cause vasodilation in the coronary arteries but vasoconstriction in the other arteries of the body.
- Myocardial infarctions are the result of ruptured artherosclerotic plaques or arterial thrombosis, which cause the death of heart tissue from prolonged ischemia.
Key Terms
- myocardial infarction: Necrosis of heart muscle caused by an interruption to the supply of blood to the heart, often as a result of prolonged ischemia.
- ischemia: Oxygen deprivation in tissues due to mechanical obstruction of the blood supply, such as by a narrowed or blocked artery or clot.
- angina: Chest pain that indicates ischemia in the heart. It may be either transient (unstable) or stable, and stable anginas typically lead to infarction.
Examples
Atherosclerotic plaques in a coronary artery will slowly occlude (block) the vessel. As the vessel diameter narrows, less blood and oxygen will pass through and a region of the myocardium will consequently not receive an adequate supply of oxygen. This could result in angina and ultimately a myocardial infarction.
Coronary circulation is the circulation of blood in the blood vessels of the heart muscle. The vessels that deliver oxygen-rich blood to the myocardium are known as coronary arteries. The vessels that remove the deoxygenated blood from the heart muscle are known as cardiac veins. The blood supply to the heart is greater than that of other body tissues since the heart has a constant metabolic demand that must be satisfied to keep the heart pumping at all times.
Coronary Circulation: Coronary arteries labeled in red text and other landmarks in blue text.
Coronary Artery Structure
The coronary arteries originate from the left side of the heart descending from the aorta. There are multiple coronary arteries derived from the larger right and left coronary arteries. For example, important coronary arteries that branch off from the larger arteries include the left anterior descending (LAD) coronary and the right posterior coronary.
Coronary arteries run both along the surface of the heart and deep within the myocardium, which has the greatest metabolic demands of all the heart tissues due to its muscle content. Epicardial coronary arteries, which run along on the surface of the heart, are capable of autoregulating vasodilation and vasoconstriction to maintain coronary blood flow at appropriate levels to fit the metabolic demands of the heart muscle. These vessels are relatively narrow and thus vulnerable to blockage, which may cause a myocardial infarction. Subendocardial coronary arteries run deep within the myocardium to provide oxygen throughout the muscle tissue of the cardiac wall.
Systole and Diastole
In systole, the ventricular myocardium contracts, generating high intraventricular pressure and compressing the subendocardial coronary vessels while allowing the epicardial coronary vessels to remain fully open. With the subendocardial coronary vessels compressed, blood flow essentially stops below the surface of the myocardium.
In diastole, the ventricular myocardium contracts, lowering the intraventricular pressure and allowing the subendocardial vessels to become open again. Due to the high pressures generated in the ventricular myocardium during systole, most myocardial tissue perfusion occurs during diastole. Additionally, catecholamines such as norephinephrine, which normally cause vasoconstriction will instead cause vasodilation within the coronary arteries. This mechanism is due to beta-adrenergic receptors in the coronary arteries and helps enable the increased cardiac output associated with fight-or-flight responses.
Myocardial Infarctions
A myocardial infarction (heart attack) may be caused by prolonged ischemia (oxygen deprivation) in the heart, which occurs due to blockage of any of the coronary arteries. Since there is very little unnecessary blood supply to the myocardium, blockage of these vessels can cause serious damage. When these vessels become blocked, the myocardium becomes oxygen-deprived, a condition called ischemia. Brief periods of ischemia in the heart are associated with intense chest pain called angina, which may either be transient if the clot breaks up on its own or stable if it does not. As the time period of ischemia increases, the hypoxic conditions cause muscle tissue to die, causing a myocardial infarction (heart attack).
Myocardial infarction is one of the most common causes of death worldwide. The clots that cause the infarction are usually the result of ruptured atherosclerotic plaques that break off and occlude the coronary arteries, but arterial thrombosis from injury or pooled blood may also cause a heart attack. The tissues of the heart do not regenerate, so those that survive a myocardial infarction will generally have scar tissue in their myocardium and may be more susceptible to other heart problems in the future.
Operation of Atrioventricular Valves
The atrioventricular valves separate the atria from the ventricles and prevent backflow from the ventricles into the atria during systole.
Learning Objectives
Describe the operation of the atrioventricular valves: bicuspid (mitral) and tricuspid
Key Takeaways
Key Points
- The atrioventricular valves, the bicuspid (mitral) and tricuspid valves, separate the atria from the ventricles.
- The bicuspid valve is on the left side of the heart and the tricuspid valve is on the right side of the heart.
- Blood flows through an atrioventricular (AV) valve when blood pressure in the atria becomes high during atrial systole and blood pressure in the ventricles becomes low enough during ventricular diastole, creating a blood pressure gradient.
- Papillary muscles, finger-like projections from the wall of the ventricles, connect the chordae tendineae (heartstrings) to the cusps of the atrioventricular valves. This connection prevents the valve from prolapsing under pressure.
- Papillary muscles, together with the chordae tendineae, make up the subvalvular apparatus.
Key Terms
- Atrioventricular valves: These valves separate the atria from the ventricles on each side of the heart and prevent backflow from the ventricles into the atria during systole. They include the mitral and tricuspid valves.
- Subvalvular apparatus: The papillary muscles and the chordae tendineae, known as the subvalvular apparatus, hold the valves closed so that they do not prolapse.
- mitral valve: The bicuspid valve that divides the left atrium and left ventricle of the heart
A heart valve allows blood flow in only one direction through the heart, and the combination of the atrioventricular and semi-lunar heart valves determines the pathway of blood flow. Valves open or close based on pressure differences across the valve. The atrioventricular (AV) valves separate the atria from the ventricles on each side of the heart and prevent backflow of blood from the ventricles into the atria during systole.
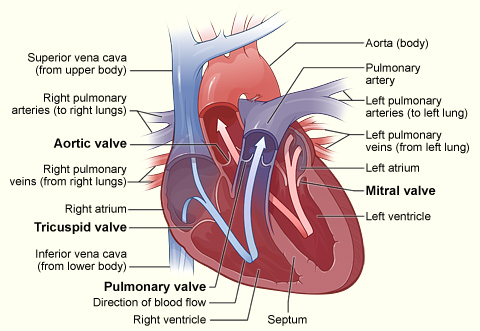
Cross section of heart indicating heart valves: The four valves determine the pathway of blood flow (indicated by arrows) through the heart
Subvalvular Apparatus
The subvalvular apparatus describes the structures beneath the AV valves that prevent the valves prom prolapsing. Valve prolapse means that the valves do not close properly, which may cause regurgitation or backflow of blood from the ventricle back into the atria, which is inefficient. The subvalvular apparatus includes the chordae tendineae and the papillary muscles. The AV valves are anchored to the wall of the ventricle by chordae tendineae (heartstrings), small tendons that prevent backflow by stopping the valve leaflets from inverting. The chordae tendineae are inelastic and attached at one end to the papillary muscles and at the other end to the valve cusps.
Papillary muscles are finger-like projections from the wall of the ventricle that anchor the chordae tendineae. This connection provides tension to hold the valves in place and prevent them from prolapsing into the atria when they close, preventing the risk of regurgitation. The subvalvular apparatus has no effect on the opening and closing of the valves, which is caused entirely by the pressure gradient of blood across the valve as blood flows from high pressure to low pressure areas.
The Mitral Valve
The mitral valve is on the left side of the heart and allows the blood to flow from the left atrium into the left ventricle. It is also known as the bicuspid valve because it contains two leaflets (cusps). The relaxation of the ventricular myocardium and the contraction of the atrial myocardium cause a pressure gradient that allows for rapid blood flow from the left atrium into the left ventricle across the mitral valve. Atrial systole (contraction) increases the pressure in the atria, while ventricular diastole (relaxation) decreases the pressure in the ventricle, causing pressure-induced flow of blood across the valve. The mitral annulus, a ring around the mitral valve, changes in shape and size during the cardiac cycle to prevent backflow. The ring contracts at the end of atrial systole due to the contraction of the left atrium around it, which aids in bringing the leaflets together to provide firm closure during ventricular systole.
The Tricuspid Valve
The tricuspid valve is the three-leaflet valve on the right side of the heart between the right atrium and the right ventricle and stops the backflow of blood between the two. The tricuspid valve functions similarly to the bicuspid valve except that three chordae tendineae connect the cusps of the valve to three papillary muscles, rather than the pair that connects the bicuspid valve. Blood passes through the tricuspid valve the same as it does through the bicuspid valve, based on a pressure gradient from high pressure to low pressure during systole and diastole.
The reason that the valves have different numbers of leaflets is not fully understood, but may arise from differences in tissue structure and pressure that occur during fetal development.
Operation of Semilunar Valves
The semilunar valves allow blood to be pumped into the major arteries while preventing backflow of blood from the arteries into the ventricles.
Learning Objectives
Describe the operation of the semilunar valves
Key Takeaways
Key Points
- The semilunar valves act to prevent backflow of blood from the arteries to the ventricles during ventricular diastole and help maintain pressure on the major arteries.
- The aortic semilunar valve separates the left ventricle from the opening of the aorta.
- The aortic and pulmonary valves are semilunar valves which separate the ventricles from the aorta and pulmonary artery, respectively.
- Partial pressure gradient changes during systole and diastole cause the opening and closing of the valves.
- Valve stenosis is when valves narrow and can’t open fully, while regurgitation is when they cannot close completely. In both instances, the heart must work harder to compensate for the faulty valves.
Key Terms
- semilunar valves: Located at the base of both the trunk of the pulmonary artery and the aorta, and prevent backflow of blood from the arteries into the ventricles.
- stenosis: The narrowing of valves, which prevents them from opening completely.
The semilunar valves are located at the connections between the pulmonary artery and the right ventricle, and the aorta and the left ventricle. These valves allow blood to be pumped forward into the arteries, but prevent backflow of blood from the arteries into the ventricles. These valves do not have subvalvular apparatus and are more similar to the semilunar valves in veins and lymphatic vessels than to atrioventricular (AV) valves.
The semilunar valves act in concert with the AV valves to direct blood flow through the heart. When the atrioventricular valves are open, the semi lunar valves are shut and blood is forced into the ventricles. When the AV valves shut, the semilunar valves open, forcing blood into the aorta and pulmonary artery. The mechanism for this process depends on blood pressure gradients in the heart, which provide the force that pushes blood through the semilunar valves.
The Aortic Valve
The aortic valve separates the left ventricle from the aorta and has three cusps. During ventricular systole, pressure rises in the left ventricle. When the pressure in the left ventricle exceeds the pressure in the aorta, the aortic valve opens and blood flows from the left ventricle into the aorta. When ventricular systole ends, pressure in the left ventricle drops rapidly, and the valve closes due to a lack of pressure imposed on them from the left ventricle. Blood pressure within the aorta following systole also causes the closing of the valve. The closing of the aortic valve produces a sound that is a component of the second heart sound.
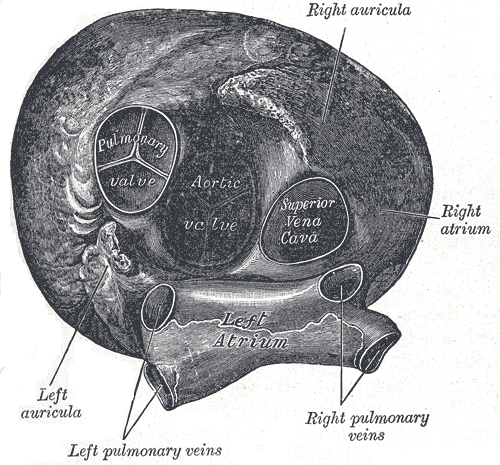
Heart viewed from above: This anterior view of the heart indicates the semilunar valves, and the aortic and pulmonary valves.
The Pulmonary Valve
The pulmonary valve (also called the pulmonic valve), which also has three cusps, separates the right ventricle from the pulmonary artery. Similar to the aortic valve, the pulmonary valve opens in ventricular systole, when the pressure in the right ventricle exceeds the pressure in the pulmonary artery. When ventricular systole ends, pressure in the right ventricle drops rapidly, and the pressure in the pulmonary artery forces the pulmonary valve to close. The closure of the pulmonary valve also produces a sound, however it is softer than the aortic sound because the blood pressure in the right side of the heart is lower compared to the left side, due to the differences between pulmonary and systemic circulation.
Valve Problems
Valves are vulnerable to several conditions that impair their normal functions. Two of the most common problems with the semilunar valves are stenosis and regurgitation. Valve stenosis refers to narrowing of the valves, which prevents the valve from opening fully, causing an obstruction of blood flow. Valve stenosis is often caused by calcium buildup and scarring from rheumatic fever, and may cause cardiac hypertrophy and heart failure. Valve regurgitation is backflow through the valves that occurs when when they cannot close completely. It is the cause of most heart murmurs, and is generally a minor problem, but if severe enough, it can cause heart failure. Stenosis and regurgitation can occur in both the semilunar and atrioventricular valves.
Systemic and Pulmonary Circulation
The cardiovascular system has two distinct circulatory paths, pulmonary circulation and systemic circulation.
Learning Objectives
Distinguish between the systemic and pulmonary circulation circuits
Key Takeaways
Key Points
- The cardiovascular system is composed of two circulatory paths: pulmonary circulation, the circuit through the lungs where blood is oxygenated, and systemic circulation, the circuit through the rest of the body to provide oxygenated blood.
- In the pulmonary circulation, blood travels through capillaries on the alveoli, air sacs in the lungs which allow for gas exchange.
- As blood flows through circulation, the size of the vessel decreases from artery / vein, to arteriole / venule, and finally to capillaries, the smallest vessels for gas and nutrient exchange.
- Systemic and pulmonary circulation transition to the opposite type of circulation when they return blood to the opposite side of the heart.
- Systemic circulation is a much larger and higher pressure system than pulmonary circulation.
Key Terms
- alveoli: Air sacs in the lungs that provide the surface for gas exchange between the air and capillaries.
- pulmonary circulation: The part of blood circulation which carries oxygen-depleted blood away from the heart, to the lungs, and returns oxygenated blood back to the heart.
- systemic circulation: The part of blood circulation that carries oxygenated blood away from the heart, to the body, and returns deoxygenated blood back to the heart.
The cardiovascular system is composed of two circulatory paths: pulmonary circulation, the circuit through the lungs where blood is oxygenated; and systemic circulation, the circuit through the rest of the body to provide oxygenated blood. The two circuits are linked to each other through the heart, creating a continuous cycle of blood through the body.
Pulmonary Circulation
Pulmonary circulation is the movement of blood from the heart to the lungs for oxygenation, then back to the heart again. Oxygen-depleted blood from the body leaves the systemic circulation when it enters the right atrium through the superior and inferior venae cavae. The blood is then pumped through the tricuspid valve into the right ventricle. From the right ventricle, blood is pumped through the pulmonary valve and into the pulmonary artery. The pulmonary artery splits into the right and left pulmonary arteries and travel to each lung.
At the lungs, the blood travels through capillary beds on the alveoli where gas exchange occurs, removing carbon dioxide and adding oxygen to the blood. Gas exchange occurs due to gas partial pressure gradients across the the alveoli of the lungs and the capillaries interwoven in the alveoli. The oxygenated blood then leaves the lungs through pulmonary veins, which returns it to the left atrium, completing the pulmonary circuit. As the pulmonary circuit ends, the systemic circuit begins.
Alveoli: A diagram of the alveoli, showing the capillary beds where gas exchange with the blood occurs.
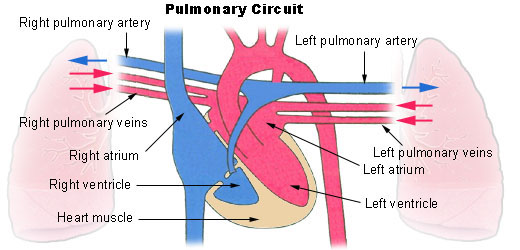
Pulmonary circuit: Diagram of pulmonary circulation. Oxygen-rich blood is shown in red; oxygen-depleted blood in blue.
Systemic Circulation
Systemic circulation is the movement of blood from the heart through the body to provide oxygen and nutrients to the tissues of the body while bringing deoxygenated blood back to the heart. Oxygenated blood enters the left atrium from the pulmonary veins. The blood is then pumped through the mitral valve into the left ventricle. From the left ventricle, blood is pumped through the aortic valve and into the aorta, the body’s largest artery. The aorta arches and branches into major arteries to the upper body before passing through the diaphragm, where it branches further into the illiac, renal, and suprarenal arteries which supply the lower parts of the body.
The arteries branch into smaller arteries, arterioles, and finally capillaries. Gas and nutrient exchange with the tissues occurs within the capillaries that run through the tissues. Metabolic waste and carbon dioxide diffuse out of the cell into the blood, while oxygen and glucose in the blood diffuses out of the blood and into the cell. Systemic circulation keeps the metabolism of every organ and every tissue in the body alive, with the exception of the parenchyma of the lungs, which are supplied by pulmonary circulation.
The deoxygenated blood continues through the capillaries which merge into venules, then veins, and finally the venae cavae, which drain into the right atrium of the heart. From the right atrium, the blood will travel through the pulmonary circulation to be oxygenated before returning gain to the system circulation, completing the cycle of circulation through the body. The arterial component of systemic circulation the highest blood pressures in the body. The venous component of systemic circulation has considerably lower blood pressure in comparison, due to their distance from the heart, but contain semi-lunar valves to compensate. Systemic circulation as a whole is a higher pressure system than pulmonary circulation simply because systemic circulation must force greater volumes of blood farther through the body compared to pulmonary circulation.