14 Organelles
The Plasma Membrane and the Cytoplasm
The plasma membrane is made up of a phospholipid bilayer that regulates the concentration of substances that can permeate a cell.
Learning Objectives
Explain the structure and purpose of the plasma membrane of a cell
Key Takeaways
Key Points
- All eukaryotic cells have a surrounding plasma membrane, which is also known as the cell membrane.
- The plasma membrane is made up by a phospholipid bilayer with embedded proteins that separates the internal contents of the cell from its surrounding environment.
- Only relatively small, non- polar materials can easily move through the lipid bilayer of the plasma membrane.
- Passive transport is the movement of substances across the membrane that does not require the use of energy while active transport is the movement of substances across the membrane using energy.
- Osmosis is the diffusion of water through a semi- permeable membrane down its concentration gradient; this occurs when there is an imbalance of solutes outside of a cell compared to the inside the cell.
Key Terms
- phospholipid: Any lipid consisting of a diglyceride combined with a phosphate group and a simple organic molecule such as choline or ethanolamine; they are important constituents of biological membranes
- hypertonic: having a greater osmotic pressure than another
- hypotonic: Having a lower osmotic pressure than another; a cell in this environment causes water to enter the cell, causing it to swell.
The Plasma Membrane
Despite differences in structure and function, all living cells in multicellular organisms have a surrounding plasma membrane (also known as the cell membrane). As the outer layer of your skin separates your body from its environment, the plasma membrane separates the inner contents of a cell from its exterior environment. The plasma membrane can be described as a phospholipid bilayer with embedded proteins that controls the passage of organic molecules, ions, water, and oxygen into and out of the cell. Wastes (such as carbon dioxide and ammonia) also leave the cell by passing through the membrane.
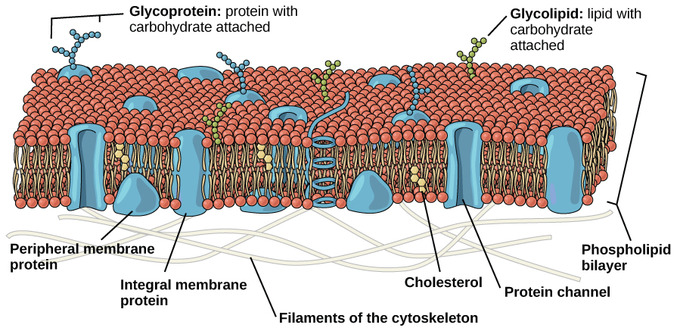
Eukaryotic Plasma Membrane: The eukaryotic plasma membrane is a phospholipid bilayer with proteins and cholesterol embedded in it.
The cell membrane is an extremely pliable structure composed primarily of two adjacent sheets of phospholipids. Cholesterol, also present, contributes to the fluidity of the membrane. A single phospholipid molecule consists of a polar phosphate “head,” which is hydrophilic, and a non-polar lipid “tail,” which is hydrophobic. Unsaturated fatty acids result in kinks in the hydrophobic tails. The phospholipid bilayer consists of two phospholipids arranged tail to tail. The hydrophobic tails associate with one another, forming the interior of the membrane. The polar heads contact the fluid inside and outside of the cell.
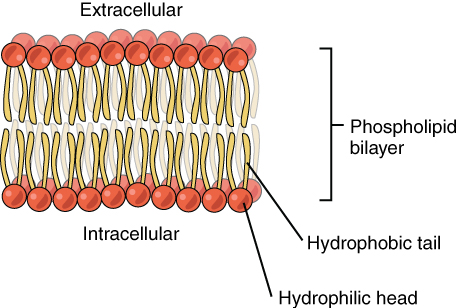
Phospholipid Bilayer: The phospholipid bilayer consists of two adjacent sheets of phospholipids, arranged tail to tail. The hydrophobic tails associate with one another, forming the interior of the membrane. The polar heads contact the fluid inside and outside of the cell.
The plasma membrane’s main function is to regulate the concentration of substances inside the cell. These substances include ions such as Ca++, Na+, K+, and Cl–; nutrients including sugars, fatty acids, and amino acids; and waste products, particularly carbon dioxide (CO2), which must leave the cell.
The membrane’s lipid bilayer structure provides the cell with access control through permeability. The phospholipids are tightly packed together, while the membrane has a hydrophobic interior. This structure causes the membrane to be selectively permeable. A membrane that has selective permeability allows only substances meeting certain criteria to pass through it unaided. In the case of the plasma membrane, only relatively small, non-polar materials can move through the lipid bilayer (remember, the lipid tails of the membrane are nonpolar). Some examples of these materials are other lipids, oxygen and carbon dioxide gases, and alcohol. However, water-soluble materials—such as glucose, amino acids, and electrolytes—need some assistance to cross the membrane because they are repelled by the hydrophobic tails of the phospholipid bilayer.
Transport Across the Membrane
All substances that move through the membrane do so by one of two general methods, which are categorized based on whether or not energy is required. Passive (non-energy requiring) transport is the movement of substances across the membrane without the expenditure of cellular energy. During this type of transport, materials move by simple diffusion or by facilitated diffusion through the membrane, down their concentration gradient. Water passes through the membrane in a diffusion process called osmosis. Osmosis is the diffusion of water through a semi-permeable membrane down its concentration gradient. It occurs when there is an imbalance of solutes outside of a cell versus inside the cell. The solution that has the higher concentration of solutes is said to be hypertonic and the solution that has the lower concentration of solutes is said to be hypotonic. Water molecules will diffuse out of the hypotonic solution and into the hypertonic solution (unless acted upon by hydrostatic forces).
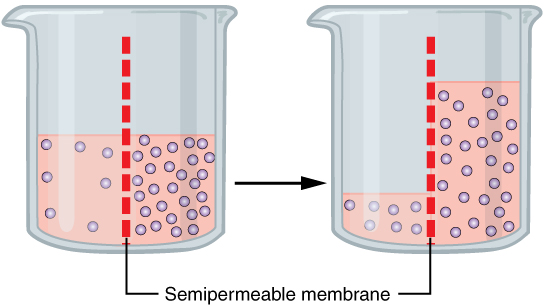
Osmosis: Osmosis is the diffusion of water through a semipermeable membrane down its concentration gradient. If a membrane is permeable to water, though not to a solute, water will equalize its own concentration by diffusing to the side of lower water concentration (and thus the side of higher solute concentration). In the beaker on the left, the solution on the right side of the membrane is hypertonic.
In contrast to passive transport, active (energy-requiring) transport is the movement of substances across the membrane using energy from adenosine triphosphate (ATP). The energy is expended to assist material movement across the membrane in a direction against their concentration gradient. Active transport may take place with the help of protein pumps or through the use of vesicles. Another form of this type of transport is endocytosis, where a cell envelopes extracellular materials using its cell membrane. The opposite process is known as exocytosis. This is where a cell exports material using vesicular transport.
Cytoplasm
The cell’s plasma membrane also helps contain the cell’s cytoplasm, which provides a gel-like environment for the cell’s organelles. The cytoplasm is the location for most cellular processes, including metabolism, protein folding, and internal transportation.
The Endoplasmic Reticulum
The endoplasmic reticulum is an organelle that is responsible for the synthesis of lipids and the modification of proteins.
Learning Objectives
Describe the structure of the endoplasmic reticulum and its role in synthesis and metabolism
Key Takeaways
Key Points
- If the endoplasmic reticulum (ER) has ribosomes attached to it, it is called rough ER; if it does not, then it is called smooth ER.
- The proteins made by the rough endoplasmic reticulum are for use outside of the cell.
- Functions of the smooth endoplasmic reticulum include synthesis of carbohydrates, lipids, and steroid hormones; detoxification of medications and poisons; and storage of calcium ions.
Key Terms
- lumen: The cavity or channel within a tube or tubular organ.
- reticulum: A network
The Endoplasmic Reticulum
The endoplasmic reticulum (ER) is a series of interconnected membranous sacs and tubules that collectively modifies proteins and synthesizes lipids. However, these two functions are performed in separate areas of the ER: the rough ER and the smooth ER. The hollow portion of the ER tubules is called the lumen or cisternal space. The membrane of the ER, which is a phospholipid bilayer embedded with proteins, is continuous with the nuclear envelope.
Rough ER
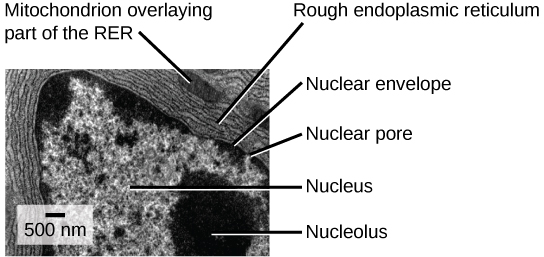
Rough Endoplasmic Reticulum: This transmission electron micrograph shows the rough endoplasmic reticulum and other organelles in a pancreatic cell.
The rough endoplasmic reticulum (RER) is so named because the ribosomes attached to its cytoplasmic surface give it a studded appearance when viewed through an electron microscope. Ribosomes transfer their newly synthesized proteins into the lumen of the RER where they undergo structural modifications, such as folding or the acquisition of side chains. These modified proteins will be incorporated into cellular membranes—the membrane of the ER or those of other organelles —or secreted from the cell (such as protein hormones, enzymes ). The RER also makes phospholipids for cellular membranes. If the phospholipids or modified proteins are not destined to stay in the RER, they will reach their destinations via transport vesicles that bud from the RER’s membrane. Since the RER is engaged in modifying proteins (such as enzymes, for example) that will be secreted from the cell, the RER is abundant in cells that secrete proteins. This is the case with cells of the liver, for example.
Smooth ER
The smooth endoplasmic reticulum (SER) is continuous with the RER but has few or no ribosomes on its cytoplasmic surface. Functions of the SER include synthesis of carbohydrates, lipids, and steroid hormones; detoxification of medications and poisons; and storage of calcium ions. In muscle cells, a specialized SER called the sarcoplasmic reticulum is responsible for storage of the calcium ions that are needed to trigger the coordinated contractions of the muscle cells.
The Golgi Apparatus
The Golgi apparatus sorts and packages materials before they leave the cell to ensure they arrive at the proper destination.
Learning Objectives
Describe the structure of the Golgi apparatus and its role in protein modification and secretion
Key Takeaways
Key Points
- The Golgi apparatus is a series of flattened sacs that sort and package cellular materials.
- The Golgi apparatus has a cis face on the ER side and a trans face opposite of the ER.
- The trans face secretes the materials into vesicles, which then fuse with the cell membrane for release from the cell.
Key Terms
- vesicle: A membrane-bound compartment found in a cell.
The Golgi Apparatus
We have already mentioned that vesicles can bud from the ER and transport their contents elsewhere, but where do the vesicles go? Before reaching their final destination, the lipids or proteins within the transport vesicles still need to be sorted, packaged, and tagged so that they wind up in the right place. Sorting, tagging, packaging, and distribution of lipids and proteins takes place in the Golgi apparatus (also called the Golgi body), a series of flattened membranes.
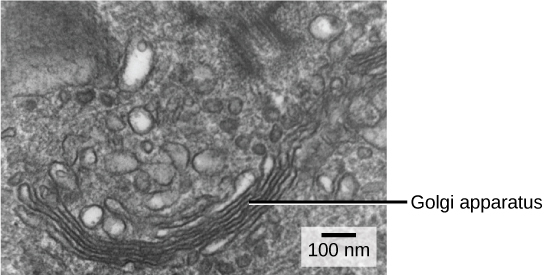
The Golgi apparatus sorts and packages cellular products: The Golgi apparatus in this white blood cell is visible as a stack of semicircular, flattened rings in the lower portion of the image. Several vesicles can be seen near the Golgi apparatus.
The receiving side of the Golgi apparatus is called the cis face. The opposite side is called the trans face. The transport vesicles that formed from the ER travel to the cis face, fuse with it, and empty their contents into the lumen of the Golgi apparatus. As the proteins and lipids travel through the Golgi, they undergo further modifications that allow them to be sorted. The most frequent modification is the addition of short chains of sugar molecules. These newly-modified proteins and lipids are then tagged with phosphate groups or other small molecules so that they can be routed to their proper destinations.
Finally, the modified and tagged proteins are packaged into secretory vesicles that bud from the trans face of the Golgi. While some of these vesicles deposit their contents into other parts of the cell where they will be used, other secretory vesicles fuse with the plasma membrane and release their contents outside the cell.
In another example of form following function, cells that engage in a great deal of secretory activity (such as cells of the salivary glands that secrete digestive enzymes or cells of the immune system that secrete antibodies) have an abundance of Golgi. In plant cells, the Golgi apparatus has the additional role of synthesizing polysaccharides, some of which are incorporated into the cell wall and some of which are used in other parts of the cell.
Lysosomes
Lysosomes are organelles that digest macromolecules, repair cell membranes, and respond to foreign substances entering the cell.
Learning Objectives
Describe how lysosomes function as the cell’s waste disposal system
Key Takeaways
Key Points
- Lysosomes breakdown/digest macromolecules (carbohydrates, lipids, proteins, and nucleic acids), repair cell membranes, and respond against foreign substances such as bacteria, viruses and other antigens.
- Lysosomes contain enzymes that break down the macromolecules and foreign invaders.
- Lysosomes are composed of lipids and proteins, with a single membrane covering the internal enzymes to prevent the lysosome from digesting the cell itself.
- Lysosomes are found in all animal cells, but are rarely found within plant cells due to the tough cell wall surrounding a plant cell that keeps out foreign substances.
Key Terms
- enzyme: a globular protein that catalyses a biological chemical reaction
- lysosome: An organelle found in all types of animal cells which contains a large range of digestive enzymes capable of splitting most biological macromolecules.
A lysosome has three main functions: the breakdown/digestion of macromolecules (carbohydrates, lipids, proteins, and nucleic acids), cell membrane repairs, and responses against foreign substances such as bacteria, viruses and other antigens. When food is eaten or absorbed by the cell, the lysosome releases its enzymes to break down complex molecules including sugars and proteins into usable energy needed by the cell to survive. If no food is provided, the lysosome’s enzymes digest other organelles within the cell in order to obtain the necessary nutrients.
In addition to their role as the digestive component and organelle-recycling facility of animal cells, lysosomes are considered to be parts of the endomembrane system. Lysosomes also use their hydrolytic enzymes to destroy pathogens (disease-causing organisms) that might enter the cell. A good example of this occurs in a group of white blood cells called macrophages, which are part of your body’s immune system. In a process known as phagocytosis or endocytosis, a section of the plasma membrane of the macrophage invaginates (folds in) and engulfs a pathogen. The invaginated section, with the pathogen inside, then pinches itself off from the plasma membrane and becomes a vesicle. The vesicle fuses with a lysosome. The lysosome’s hydrolytic enzymes then destroy the pathogen.
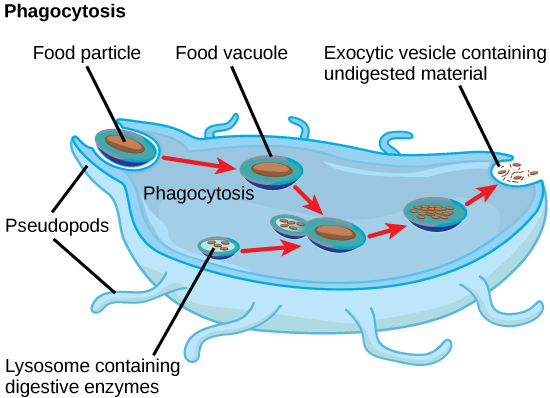
Lysosomes digest foreign substances that might harm the cell: A macrophage has engulfed (phagocytized) a potentially pathogenic bacterium and then fuses with a lysosomes within the cell to destroy the pathogen. Other organelles are present in the cell but for simplicity are not shown.
A lysosome is composed of lipids, which make up the membrane, and proteins, which make up the enzymes within the membrane. Usually, lysosomes are between 0.1 to 1.2μm, but the size varies based on the cell type. The general structure of a lysosome consists of a collection of enzymes surrounded by a single-layer membrane. The membrane is a crucial aspect of its structure because without it the enzymes within the lysosome that are used to breakdown foreign substances would leak out and digest the entire cell, causing it to die.
Lysosomes are found in nearly every animal-like eukaryotic cell. They are so common in animal cells because, when animal cells take in or absorb food, they need the enzymes found in lysosomes in order to digest and use the food for energy. On the other hand, lysosomes are not commonly-found in plant cells. Lysosomes are not needed in plant cells because they have cell walls that are tough enough to keep the large/foreign substances that lysosomes would usually digest out of the cell.
Peroxisomes
Peroxisomes neutralize harmful toxins and carry out lipid metabolism and oxidation reactions that break down fatty acids and amino acids.
Learning Objectives
Name the various functions that peroxisomes perform inside the cell
Key Takeaways
Key Points
- Lipid metabolism and chemical detoxification are important functions of peroxisomes.
- Peroxisomes are responsible for oxidation reactions that break down fatty acids and amino acids.
- Peroxisomes oversee reactions that neutralize free radicals, which cause cellular damage and cell death.
- Peroxisomes chemically neutralize poisons through a process that produces large amounts of toxic H2O2, which is then converted into water and oxygen.
- The liver is the organ primarily responsible for detoxifying the blood before it travels throughout the body; as a result, liver cells contain large amounts of peroxisomes.
Key Terms
- enzyme: a globular protein that catalyses a biological chemical reaction
- free radical: Any molecule, ion or atom that has one or more unpaired electrons; they are generally highly reactive and often only occur as transient species.
Peroxisomes
A type of organelle found in both animal cells and plant cells, a peroxisome is a membrane-bound cellular organelle that contains mostly enzymes. Peroxisomes perform important functions, including lipid metabolism and chemical detoxification. They also carry out oxidation reactions that break down fatty acids and amino acids.
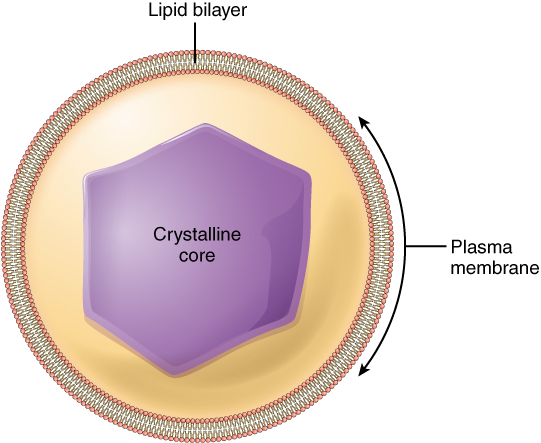
Peroxisomes: Peroxisomes are membrane-bound organelles that contain an abundance of enzymes for detoxifying harmful substances and lipid metabolism.
In contrast to the digestive enzymes found in lysosomes, the enzymes within peroxisomes serve to transfer hydrogen atoms from various molecules to oxygen, producing hydrogen peroxide (H2O2). In this way, peroxisomes neutralize poisons, such as alcohol, that enter the body. In order to appreciate the importance of peroxisomes, it is necessary to understand the concept of reactive oxygen species.
Reactive oxygen species (ROS), such as peroxides and free radicals, are the highly-reactive products of many normal cellular processes, including the mitochondrial reactions that produce ATP and oxygen metabolism. Examples of ROS include the hydroxyl radical OH, H2O2, and superoxide (O−2). Some ROS are important for certain cellular functions, such as cell signaling processes and immune responses against foreign substances. Many ROS, however, are harmful to the body. Free radicals are reactive because they contain free unpaired electrons; they can easily oxidize other molecules throughout the cell, causing cellular damage and even cell death. Free radicals are thought to play a role in many destructive processes in the body, from cancer to coronary artery disease.
Peroxisomes oversee reactions that neutralize free radicals. They produce large amounts of the toxic H2O2 in the process, but contain enzymes that convert H2O2 into water and oxygen. These by-products are then safely released into the cytoplasm. Like miniature sewage treatment plants, peroxisomes neutralize harmful toxins so that they do not cause damage in the cells. The liver is the organ primarily responsible for detoxifying the blood before it travels throughout the body; liver cells contain an exceptionally high number of peroxisomes.
Mitochondria
Mitochondria are organelles that are responsible for making adenosine triphosphate (ATP), the cell’s main energy-carrying molecule.
Learning Objectives
Explain the role of the mitochondria.
Key Takeaways
Key Points
- Mitochondria contain their own ribosomes and DNA; combined with their double membrane, these features suggest that they might have once been free-living prokaryotes that were engulfed by a larger cell.
- Mitochondria have an important role in cellular respiration through the production of ATP, using chemical energy found in glucose and other nutrients.
- Mitochondria are also responsible for generating clusters of iron and sulfur, which are important cofactors of many enzymes.
Key Terms
- alpha-proteobacteria: A taxonomic class within the phylum Proteobacteria — the phototropic proteobacteria.
- adenosine triphosphate: a multifunctional nucleoside triphosphate used in cells as a coenzyme, often called the “molecular unit of energy currency” in intracellular energy transfer
- cofactor: an inorganic molecule that is necessary for an enzyme to function
One of the major features distinguishing prokaryotes from eukaryotes is the presence of mitochondria. Mitochondria are double-membraned organelles that contain their own ribosomes and DNA. Each membrane is a phospholipid bilayer embedded with proteins. Eukaryotic cells may contain anywhere from one to several thousand mitochondria, depending on the cell’s level of energy consumption. Each mitochondrion measures 1 to 10 micrometers (or greater) in length and exists in the cell as an organelle that can be ovoid to worm-shaped to intricately branched.
Mitochondria Structure
Most mitochondria are surrounded by two membranes, which would result when one membrane-bound organism was engulfed into a vacuole by another membrane-bound organism. The mitochondrial inner membrane is extensive and involves substantial infoldings called cristae that resemble the textured, outer surface of alpha-proteobacteria. The matrix and inner membrane are rich with the enzymes necessary for aerobic respiration.
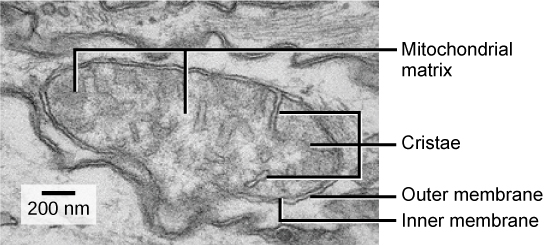
Mitochondrial structure: This electron micrograph shows a mitochondrion as viewed with a transmission electron microscope. This organelle has an outer membrane and an inner membrane. The inner membrane contains folds, called cristae, which increase its surface area. The space between the two membranes is called the intermembrane space, and the space inside the inner membrane is called the mitochondrial matrix. ATP synthesis takes place on the inner membrane.
Mitochondria have their own (usually) circular DNA chromosome that is stabilized by attachments to the inner membrane and carries genes similar to genes expressed by alpha-proteobacteria. Mitochondria also have special ribosomes and transfer RNAs that resemble these components in prokaryotes. These features all support the hypothesis that mitochondria were once free-living prokaryotes.
Mitochondria Function
Mitochondria are often called the “powerhouses” or “energy factories” of a cell because they are responsible for making adenosine triphosphate (ATP), the cell’s main energy-carrying molecule. ATP represents the short-term stored energy of the cell. Cellular respiration is the process of making ATP using the chemical energy found in glucose and other nutrients. In mitochondria, this process uses oxygen and produces carbon dioxide as a waste product. In fact, the carbon dioxide that you exhale with every breath comes from the cellular reactions that produce carbon dioxide as a by-product.
It is important to point out that muscle cells have a very high concentration of mitochondria that produce ATP. Your muscle cells need a lot of energy to keep your body moving. When your cells don’t get enough oxygen, they do not make a lot of ATP. Instead, the small amount of ATP they make in the absence of oxygen is accompanied by the production of lactic acid.
In addition to the aerobic generation of ATP, mitochondria have several other metabolic functions. One of these functions is to generate clusters of iron and sulfur that are important cofactors of many enzymes. Such functions are often associated with the reduced mitochondrion-derived organelles of anaerobic eukaryotes.
Origins of Mitochondria
There are two hypotheses about the origin of mitochondria: endosymbiotic and autogenous, but the most accredited theory at present is endosymbiosis. The endosymbiotic hypothesis suggests mitochondria were originally prokaryotic cells, capable of implementing oxidative mechanisms. These prokaryotic cells may have been engulfed by a eukaryote and became endosymbionts living inside the eukaryote.