225 Development of the Nervous System
Development of the Central Nervous System
The central nervous system (CNS) develops from a longitudinal groove on the neural plate that forms the rudimentary nervous system.
Learning Objectives
Describe the development of the central nervous system
Key Takeaways
Key Points
- During early development of the vertebrate embryo, a longitudinal groove on the neural plate gradually deepens and the ridges on either side of it (the neural folds) ultimately meet, transforming it into a closed tube, the ectodermal wall of which forms the rudimentary nervous system.
- This tube initially differentiates into three vesicles (pockets): the prosencephalon at the front, the mesencephalon,
the rhombencephalon
between the mesencephalon and spinal cord. - The telencephalon differentiates into, among other things, the striatum, the hippocampus, and the neocortex, and its cavity becomes the first and second ventricles. Diencephalon elaborations include the subthalamus, hypothalamus, thalamus, and epithalamus, and its cavity forms the third ventricle.
- The survival of neurons is regulated by survival factors, called trophic factors.
- Agrin is not a central mediator of CNS synapse formation and there is active interest in identifying signals that mediate CNS synaptogenesis.
Key Terms
- diencephalon: The region of the human brain, specifically the forebrain, that includes the thalamus, hypothalamus, epithalamus, prethalamus or subthalamus, and pretectum.
- metencephalon: The portion of the embryonic rhombencephalon from which the pons and cerebellum arise.
- telencephalon: The anterior part of the forebrain; the endbrain.
The central nervous system (CNS) consists of the brain and the spinal cord. It is the part of the nervous system that integrates the information that it receives from, and coordinates the activity of, all parts of the bodies of bilaterian animals—that is, all multicellular animals except radially symmetric animals such as sponges and jellyfish.
Some classifications of the CNS also include the retina and the cranial nerves. Together with the peripheral nervous system, it has a fundamental role in behavior control. The CNS is contained within the dorsal cavity, with the brain in the cranial cavity and the spinal cord in the spinal cavity. In vertebrates, the brain is protected by the skull while the spinal cord is protected by the vertebrae, and both are enclosed in the meninges.
During early development of the vertebrate embryo, a longitudinal groove on the neural plate gradually deepens and the ridges on either side of it (the neural folds) become elevated and ultimately meet, transforming the groove into a closed tube, the ectodermal wall of which forms the rudiment of the nervous system. This tube initially differentiates into three vesicles (pockets): the prosencephalon at the front, the mesencephalon, and between the mesencephalon and the spinal cord, the rhombencephalon. At six weeks in human embryo development, the prosencephalon divides further into the telencephalon and diencephalon and the rhombencephalon divides into the metencephalon and myelencephalon.
As the vertebrate grows, these vesicles differentiate further still. The telencephalon differentiates into, among other things, the striatum, the hippocampus, and the neocortex, and its cavity becomes the first and second ventricles. Diencephalon elaborations include the subthalamus, hypothalamus, thalamus, and epithalamus, and its cavity forms the third ventricle. The tectum, pretectum, cerebral peduncle, and other structures develop out of the mesencephalon, and its cavity grows into the mesencephalic duct (cerebral aqueduct). The metencephalon becomes, among other things, the pons and the cerebellum; the myelencephalon forms the medulla oblongata; and their cavities develop into the fourth ventricle.
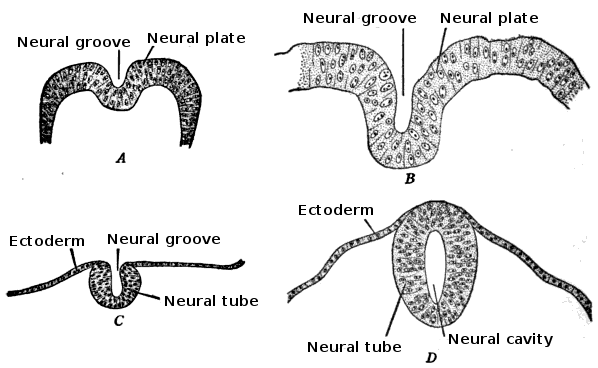
Morphogenisis and the Change in the Shape of Cell: Development of the neural tube in human embryos (Prentiss-Arey). A: An early embryo (Keibel) B: At 2 mm. (Graf Spee) C: At 2 mm. (Mall) D: At 2.7 mm (Kollmann).
Development of the Peripheral Nervous System
The peripheral nervous system develops from two strips of tissue called the neural crest, running lengthwise above the neural tube.
Learning Objectives
Describe peripheral nervous system development
Key Takeaways
Key Points
- The first sign of the nervous system is the appearance of a thin strip of cells along the center of the back, called the neural plate. The inner portion of the neural plate is destined to become the central nervous system (CNS), while the outer portion will become the peripheral nervous system (PNS).
- Neurulation (neural development) progresses with the formation of the neural groove which closes to form the neural tube and neural crest.
- Neural crest cells from the roof plate of the neural tube migrate through the periphery where they differentiate into varied cell types, including pigment cells and the cells of the PNS.
Key Terms
- neurulation: The process by which the beginnings of the vertebrate nervous system is formed in embryos.
- neural plate: A thick, flat bundle of ectoderm formed in vertebrate embryos after induction by the notochord.
- neural crest: A strip of ectodermal material in the early vertebrate embryo inserted between the prospective neural plate and the epidermis.
In vertebrates, the first sign of the nervous system is the appearance of a thin strip of cells along the center of the back, called the neural plate. The inner portion of the neural plate (along the midline) is destined to become the central nervous system (CNS), the outer portion the peripheral nervous system (PNS). As development proceeds, a fold called the neural groove appears along the midline. This fold deepens and then closes up at the top. At this point the future CNS appears as a cylindrical structure called the neural tube, whereas the future PNS appears as two strips of tissue called the neural crest, running lengthwise above the neural tube. The sequence of stages from neural plate to neural tube and neural crest is known as neurulation.
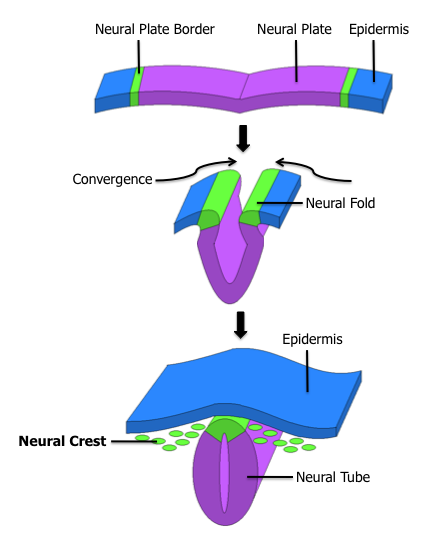
Formation of the Fetal Nervous System: The neural tube will give rise to the central nervous system, while the neural crest will give rise to the peripheral nervous system. This diagram shows the epidermis, neural fold, and convergence.
Neural crest cells are a transient, multipotent, migratory cell population unique to vertebrates that gives rise to a diverse cell lineage including melanocytes, craniofacial cartilage and bone, smooth muscle, peripheral and enteric neurons and glia. After gastrulation, neural crest cells are specified at the border of the neural plate and the non-neural ectoderm. During neurulation, the borders of the neural plate, also known as the neural folds, converge at the dorsal midline to form the neural tube. Subsequently, neural crest cells from the roof plate of the neural tube undergo an epithelial to mesenchymal transition, delaminating from the neuroepithelium and migrating through the periphery where they differentiate into varied cell types, including pigment cells and the cells of the PNS. The emergence of the neural crest was important in vertebrate evolution because many of its structural derivatives are defining features of the vertebrate clade.
Regeneration of Nerve Fibers
The peripheral nervous system (PNS) is able to repair and regenerate itself, but the central nervous system is incapable of doing so.
Learning Objectives
Describe nerve fiber regeneration in the PNS
Key Takeaways
Key Points
- In the PNS, some self-regeneration after injury is possible if the cell body (soma) and portions of the membrane ( neurilemma ) are left intact.
- When an axon is damaged, the distal segment undergoes Wallerian degeneration, losing its myelin sheath. The proximal segment can either die by apoptosis or undergo the chromatolytic reaction, which is an attempt at repair.
- Injury to the PNS immediately elicits the migration of phagocytes, Schwann cells, and macrophages to the lesion site to clear away debris such as damaged tissue.
- In the case of a severed nerve, the proximal end begins to sprout axons and the presence of growth cones can be detected. Meanwhile, the distal end experiences Wallerian degeneration within hours of the injury; the axons and myelin degenerate, but the endoneurium remains.
- The environment within the CNS, especially following trauma, counteracts the repair of myelin and neurons. Glial scars rapidly form, and the glia actually produce factors that inhibit remyelination and axon repair.
Key Terms
- neurilemma: The outer membranous covering of a nerve fiber.
- microglia: The glial cells of the immune system; they can function as phagocytes.
- neuroregeneration: The regrowth or repair of damaged nervous tissue.
- Schwann cell: Glia of the peripheral nervous system, involved in many important aspects of peripheral nerve biology.
Nerve damage can be caused by physical injury or swelling (e.g. carpal tunnel syndrome), autoimmune disease (e.g. Guillain-Barré syndrome), infection (e.g. neuritis), diabetes, or failure of the blood vessels surrounding the nerve. Nerves can be easily damaged in a traumatic event, but can regenerate if the soma and a small portion of the neurilemma remain. Neuroregeneration refers to the regrowth or repair of nervous tissues, cells, or cell products. Such mechanisms may include generation of new neurons, glia, axons, myelin, or synapses. Neuroregeneration differs between the peripheral nervous system (PNS) and the central nervous system (CNS) by the functional mechanisms and especially by the extent and speed. When an axon is damaged, the distal segment undergoes Wallerian degeneration, losing its myelin sheath. The proximal segment can either die by apoptosis or undergo the chromatolytic reaction, an attempt at repair. In the CNS, synaptic stripping occurs as glia foot processes invade the dead synapse.
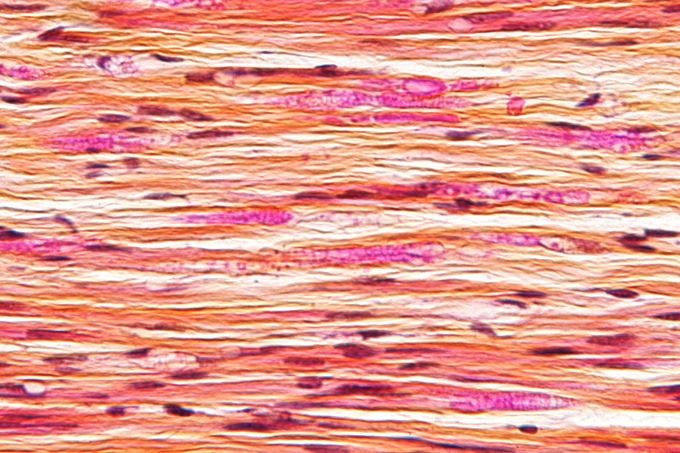
Nerve injury: Micrograph of a nerve with a decrease in myelinated nerve fibres (pink) and an abnormal increase in fibrous tissue (yellow), as may be seen in nerve injuries.
Nervous system injuries affect more than 90,000 people every year, 10,000 of which are spinal cord injuries. As a result, the field of nerve regeneration and repair, a subfield of neural tissue engineering dedicated to the discovery of new ways to recover nerve functionality after injury, is growing rapidly. The nervous system is divided into two parts: the CNS, which consists of the brain and spinal cord, and the PNS, which consists of cranial and spinal nerves along with their associated ganglia. While the PNS has an intrinsic ability for repair and regeneration, the CNS is for the most part incapable of self-repair and regeneration. There is no current treatment to recover human nerve function after injury to the central nervous system. In addition, multiple attempts at nerve re-growth across the PNS-CNS transition have not been successful. Although the PNS has the capability for regeneration, much research still needs to be done to optimize the environment for maximum regrowth potential. Nerve regeneration is part of the pathogenesis of many diseases, including multiple sclerosis.
PNS Injury
Neuroregeneration in the PNS occurs to a significant degree. Axonal sprouts form at the proximal stump and grow until they enter the distal stump. The growth of the sprouts are governed by chemotactic factors secreted from Schwann cells (neurolemmocytes). Injury to the PNS immediately elicits the migration of phagocytes, Schwann cells, and macrophages to the lesion site to clear away debris such as damaged tissue. When a nerve axon is severed, the end still attached to the cell body is labeled the proximal segment, while the other end is called the distal segment. After injury, the proximal end swells and experiences some retrograde degeneration, but once the debris is cleared, it begins to sprout axons and the presence of growth cones can be detected. The proximal axons are able to regrow as long as the cell body is intact and they have made contact with the Schwann cells in the endoneurial channel. Human axon growth rates can reach 2 mm/day in small nerves and 5 mm/day in large nerves. The distal segment, however, experiences Wallerian degeneration within hours of the injury; the axons and myelin degenerate, but the endoneurium remains. In the later stages of regeneration, the remaining endoneurial tube directs axon growth back to the correct targets. During Wallerian degeneration, Schwann cells grow in ordered columns along the endoneurial tube. This creates a band of Büngner (boB) that protects and preserves the endoneurial channel. Also, macrophages and Schwann cells release neurotrophic factors that enhance regrowth.
CNS Injury
Unlike PNS injury, injury to the CNS is not followed by extensive regeneration. It is limited by the inhibitory influences of the glial and extracellular environment. The hostile, non-permissible growth environment is in part created by the migration of myelin-associated inhibitors, astrocytes, oligodendrocytes, oligodendrocyte precursors, and microglia. The environment within the CNS, especially following trauma, counteracts the repair of myelin and neurons. Glial scars rapidly form and the glia actually produce factors that inhibit remyelination and axon repair. The axons themselves also lose the potential for growth with age. Slower degeneration of the distal segment than that which occurs in the peripheral nervous system also contributes to the inhibitory environment; inhibitory myelin and axonal debris are not cleared away as quickly. All these factors contribute to the formation of what is known as a glial scar, which axons cannot grow across.
Aging and the Nervous System
One of the effects of aging on the nervous system is the loss of neurons in the cerebral cortex.
Learning Objectives
Describe the effects of aging on the nervous system
Key Takeaways
Key Points
- As the brain ages, neurons in the central nervous system (CNS), which do not typically regenerate, are lost. This reduces the brain’s capacity to send and receive nerve impulses and slows information processing.
- Dementia is the age-related progressive decline in cognitive function. This may affect memory, attention, language, and problem-solving.
- Alzheimer’s disease is a neurodegenerative disease characterized by progressive cognitive deterioration, declining activities of daily living, and neuropsychiatric symptoms or behavioral changes. It is the most common cause of dementia.
Key Terms
- cerebral cortex: The gray, folded, outermost layer of the cerebrum that is responsible for higher brain processes such as sensation, voluntary muscle movement, thought, reasoning, and memory.
- dementia: A progressive decline in cognitive function due to brain damage or disease. Areas particularly affected include memory, attention, judgement, language, and problem solving.
Neuron loss is an effect of aging on the nervous system. By the age of 30, the brain begins to lose thousands of neurons each day, causing a decreased capacity to send nerve impulses to and from the brain and slowing information processing. In addition, voluntary motor movements slow down, reflex time increases, and conduction velocity decreases.
Dementia (from Latin de- “apart, away” + mens (genitive mentis) “mind”) is the progressive decline in cognitive function due to damage or disease in the brain beyond what is expected from normal aging. Dementia most commonly affects memory, attention, language, and problem solving, although those in the later stages in this condition may be disoriented in time, place, and person. Symptoms of dementia can be classified as either reversible or irreversible depending upon the etiology of the disease. Less than 10% of all dementia cases are reversible.
Alzheimer’s disease (AD) is a neurodegenerative disease characterized by progressive cognitive deterioration, declining activities of daily living, and neuropsychiatric symptoms or behavioral changes. It is the most common cause of dementia. The most striking early symptom is short term memory loss (amnesia), which usually manifests as minor forgetfulness that becomes steadily more pronounced with illness progression. Older memories are often preserved. As the disorder progresses, cognitive (intellectual) impairment extends to the domains of language (aphasia), skilled movements (apraxia), recognition (agnosia), and functions (such as decision-making and planning) closely related to the frontal and temporal lobes of the brain as they become disconnected from the limbic system, reflecting extension of the underlying pathological process.
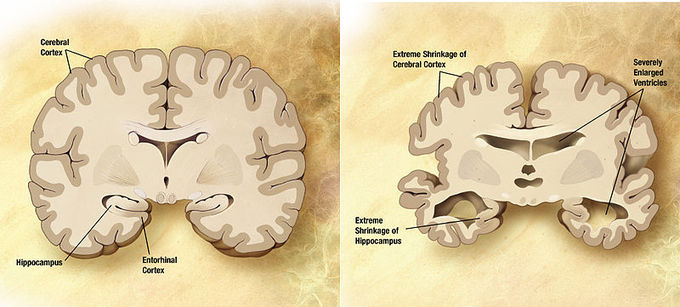
Effects of Alzheimer’s Disease: Comparison of a normal aged brain (left) and the brain of a person with Alzheimer’s (right). Differential characteristics are pointed out in the hippocampus, cerebral cortex, and ventricles.
The symptoms of Alzheimer’s are triggered by neuron loss and an inflammatory response to the deposition of amyloid plaques and neurofibrillary tangles. Alzheimer’s has a genetic component, and autosomal dominant mutations in three different genes have been identified that account for a small number of cases of familial, early-onset AD. For late onset AD (LOAD), only one susceptibility gene has so far been identified: the epsilon 4 allele of the apolipoprotein E gene. Age of onset itself has a heritability of around 50%.