29 5.2 Chemical Weathering
Chemical weathering results from chemical changes to minerals that become unstable when they are exposed to surface conditions. The kinds of changes that take place are highly specific to the mineral and the environmental conditions. Some minerals, like quartz, are virtually unaffected by chemical weathering, while others, like feldspar, are easily altered. In general, the degree of chemical weathering is greatest in warm and wet climates, and least in cold and dry climates. The important characteristics of surface conditions that lead to chemical weathering are the presence of water (in the air and on the ground surface), the abundance of oxygen, and the presence of carbon dioxide, which produces weak carbonic acid when combined with water. That process, which is fundamental to most chemical weathering, can be shown as follows:
H2O + CO2 ↔ H2CO3 then H2CO3 ↔ H+ + HCO3− water + carbon dioxide ↔ carbonic acid then carbonic acid ↔ dissolved hydrogen ions + dissolved bicarbonate ions |
Yikes! Chemical formulas
Lots of people seize up when they are asked to read chemical or mathematical formulas. It’s OK, you don’t necessarily have to! If you don’t like the formulas just read the text underneath them. In time you may get used to reading the formulas.
The double-ended arrow “↔ ” indicates that the reaction can go either way, but for our purposes these reactions are going towards the right.
Here we have water (e.g., as rain) plus carbon dioxide in the atmosphere, combining to create carbonic acid. Then carbonic acid dissociates (comes apart) to form hydrogen and bicarbonate ions. The amount of CO2 in the air is enough to make weak carbonic acid. There is typically much more CO2 in the soil, so water that percolates through the soil can become more acidic. In either case, this acidic water is a critical to chemical weathering.
In some types of chemical weathering the original mineral becomes altered to a different mineral. For example, feldspar is altered—by hydrolysis—to form clay minerals plus some ions in solution. In other cases the minerals dissolve completely, and their components go into solution. For example, calcite (CaCO3) is soluble in acidic solutions.
The hydrolysis of feldspar can be written like this:
CaAl2Si2O8 + H2CO3 + ½O2 ↔ Al2Si2O5(OH)4 + Ca2+ + CO32− plagioclase feldspar + carbonic acid ↔ kaolinite + dissolved calcium ions + dissolved carbonate ions |
This reaction shows calcium-bearing plagioclase feldspar, but similar reactions could also be written for sodium or potassium feldspars. In this case, we end up with the mineral kaolinite, along with calcium and carbonate ions in solution. Those ions can eventually combine (probably in the ocean) to form the mineral calcite. The hydrolysis of feldspar to clay is illustrated in Figure 5.2.1, which shows two images of the same granitic rock, a recently broken fresh surface on the left and a clay-altered weathered surface on the right. Other silicate minerals can also go through hydrolysis, although the end results will be a little different. For example, pyroxene can be converted to the clay minerals chlorite or smectite, and olivine can be converted to the clay mineral serpentine.

Oxidation is another very important chemical weathering process. The oxidation of the iron in a ferromagnesian silicate starts with the dissolution of the iron. For olivine, the process looks like this, where olivine in the presence of carbonic acid is converted to dissolved iron, carbonate, and silicic acid:
Fe2SiO4+ 4H2CO3 ↔ 2Fe2+ + 4HCO3− + H4SiO4 olivine + (carbonic acid) ↔ dissolved iron ions + dissolved carbonate ions + dissolved silicic acid |
But in the presence of oxygen and carbonic acid, the dissolved iron is then quickly converted to the mineral hematite:
2Fe2+ + 4HCO3− + ½ O2 + 2H2O ↔ Fe2O3 + 4H2CO3 dissolved iron ions + dissolved bicarbonate ions + oxygen + water ↔ hematite + carbonic acid |
The equation shown here is for olivine, but it could apply to almost any other ferromagnesian silicate, including pyroxene, amphibole, or biotite. Iron in the sulphide minerals (e.g., pyrite) can also be oxidized in this way. And the mineral hematite is not the only possible end result, as there is a wide range of iron oxide minerals that can form in this way. The results of this process are illustrated in Figure 5.2.2, which shows a granitic rock in which some of the biotite and amphibole have been altered to form the iron oxide mineral limonite.

A special type of oxidation takes place in areas where the rocks have elevated levels of sulphide minerals, especially pyrite (FeS2). Pyrite reacts with water and oxygen to form sulphuric acid, as follows:
2FeS2 + 7O2 + 2H2O ↔ 2Fe2+ H2SO4 + 2H+ pyrite + oxygen + water ↔ dissloved iron ions + sulphuric acid + dissolved hydrogen ions |
The runoff from areas where this process is taking place is known as acid rock drainage (ARD), and even a rock with 1% or 2% pyrite can produce significant ARD. Some of the worst examples of ARD are at metal mine sites, especially where pyrite-bearing rock and waste material have been mined from deep underground and then piled up and left exposed to water and oxygen. One example of that is the Mt. Washington Mine near Courtenay on Vancouver Island (Figure 5.2.3), but there are many similar sites across Canada and around the world.
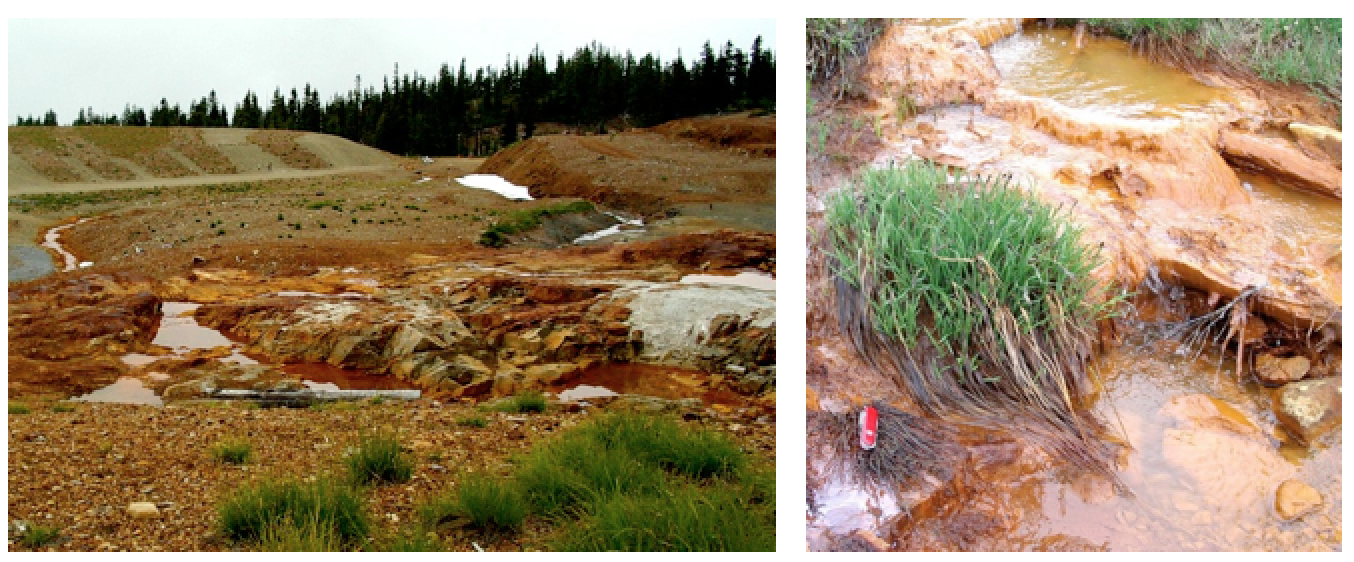
At many ARD sites, the pH of the runoff water is less than 4 (very acidic). Under these conditions, metals such as copper, zinc, and lead are quite soluble, and this can lead to toxicity for aquatic and other organisms. For many years, the river downstream from the Mt. Washington Mine had so much dissolved copper in it that it was toxic to salmon. Remediation work has since been carried out at the mine and the situation has improved.
The hydrolysis of feldspar and other silicate minerals and the oxidation of iron in ferromagnesian silicates all serve to create rocks that are softer and weaker than they were to begin with, and thus more susceptible to mechanical weathering.
The weathering reactions that we’ve discussed so far involved the transformation of one mineral to another mineral (e.g., feldspar to clay), and the release of some ions in solution (e.g., Ca2+ or Fe2+). Some weathering processes involve the complete dissolution of a mineral. Calcite, for example, will dissolve in weak acid, to produce calcium and bicarbonate ions. The equation is as follows:
CaCO3 + H+ + HCO3− ↔ Ca2+ + 2HCO3− calcite + dissolved hydrogen ions + dissolved bicarbonate ions ↔ dissolved calcium ions + dissolved bicarbonate ions |
Calcite is the major component of limestone (typically more than 95%), and under surface conditions, limestone can dissolve completely, as shown in Figure 5.2.4. Limestone also dissolves at relatively shallow depths underground, forming limestone caves. This is discussed in more detail in Chapter 14, where we look at groundwater.
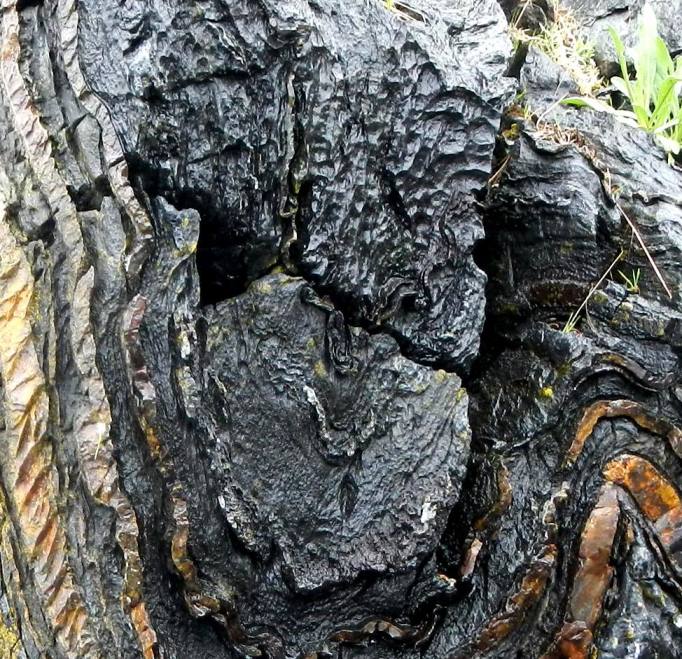
Exercise 5.2 Chemical Weathering
The main processes of chemical weathering are hydrolysis, oxidation, and dissolution. Indicate which process is primarily involved during each of the following chemical weathering changes:
- Pyrite to hematite
- Calcite to calcium and bicarbonate ions
- Feldspar to clay
- Olivine to serpentine
See Appendix 3 for Exercise 5.2 answers.
Media Attributions
- Figure 5.2.1, 5.2.2, 5.2.3, 5.2.4: © Steven Earle. CC BY.
a reaction between a mineral and water in which H+ ions are added to the mineral and a chemically equivalent amount of cations are released into solution
a hydrous sheet silicate mineral that typically exists as clay-sized grains
the reaction between a mineral and oxygen
the production of acid from oxidation of sulphide minerals (especially pyrite) in either naturally or anthropogenically exposed rock
water that lies beneath the surface of the ground